1.1.1 State that error bars are graphical representations of the variability of data |
|
Error bars are graphical representations of the variability of data. |
| |
1.1.2 Calculate the mean and standard deviation of a set of values. |
|
The mean of a set of values is calculated by dividing the sum of the values by the number of values. The standard deviation is calculated by entering the data into a calculator and using the standard deviation function button. |
| |
1.1.3 State that the term standard deviation is used to summarize the spread of values around the mean, and that 68% of the values fall within one standard deviation of the mean. |
|
The term standard deviation is used to summarize the spread of values around the mean, and that 68% of the values fall within one standard deviation of the mean. This rises to about 95% for +/- 2 standard deviations. |
| |
1.1.4 Explain how the standard deviation is useful for comparing the means and spread of data between two or more samples. |
|
The standard deviation is used to show how the values are spread above and below the mean. A low standard deviation means that the values are closely grouped around the mean whereas a high standard deviation means that the values are widely spread. About 68% of the values fall within one standard deviation of the mean. This rises to about 95% for +/- 2 standard deviations.
We can use the standard deviation to decide weather the differences between two means is significant. If the difference between the two means is larger than that of the standard deviations then the difference between the two means is significant. If the difference between the two means is smaller than that of the standard deviation then the differences between the two means are insignificant. |
| |
1.1.5 Deduce the significance of the differences between two sets of data using calculated values for t and the appropriate tables. |
|
1. enter values into calculator2. determine t3. find # of degrees of freedom4. find critical value of t and use 0.05 for P5. compare the calculated value of t with critical value |
| |
1.1.6 Explain that the existence of a correlation does not establish that there is a casual relationship between two variables. |
|
1.1.6 Explain that the existence of a correlation does not establish that there is a casual relationship between two variables. |
| |
2.1.1 Outline the cell theory. |
|
The cell theory states that:
-
All living organisms are composed of cells. Multicellular organisms (example: humans) are composed of many cells while unicellular organisms (example: bacteria) are composed of only one cell. Cells are the basic unit of structure in all organisms.
-
Cells are the smallest unit of life. They are the smallest structures capable of surviving on their own.
-
Cells come from pre-exsisting cells and cannot be created from non-living material. For example, new cells arise from cell division and a zygote (the very first cell formed when an organism is produced) arises from the fusion of an egg cell and a sperm cell. |
| |
2.1.2 Discuss the evidence for the cell theory. |
|
When scientists started to look at the structures of organisms under the microscope they discovered that all living organisms where made up of these small units which they proceeded to call cells. When these cells were taken from tissues they were able to survive for some period of time. Nothing smaller than the cell was able to live independently and so it was concluded that the cell was the smallest unit of life. For some time, scientists thought that cells must arise from non-living material but it was eventually proven that this was not the case, instead they had to arise from pre-exsisting cells. An experiment to prove this can be done as follows:
-
Take two containers and put food in both of these
-
Sterilize both of the containers so that all living organisms are killed
-
Leave one of the containers open and seal the other closed
What will happen is that in the open container mold will start to grow but in the container that was sealed no mold will be present. The reason for this is because in the open container, cells are able to enter the container from the external environment and start to divide and grow. However, due to the seal on the other container no cells will be able to enter and so no mold will develop, proving that cells cannot arise from non-living material. |
| |
2.1.3 State that unicellular organisms carry out all the functions of life. |
|
Unicellular organisms carry out all the functions of life including metabolism, response, homeostasis, growth, reproduction and nutrition. |
| |
2.1.4 Compare the relative sizes of molecules, cell membrane thickness, viruses, bacteria, organelles and cells, using the appropriate SI unit. |
|
Remember: 1 millimeter (mm) = 10-3 meters 1 micrometer (μm) = 10-3 millimeters 1 nanometer (nm) = 10-3 micrometers
A molecule = 1 nm Thickness of cell membrane = 10 nm Viruses = 100 nm Bacteria = 1μm Organelles = up to 10 μm Eukaryotic cells = up to 100 μm |
| |
2.1.5 Calculate the linear magnification of drawings and the actual size of specimens in images of known magnification. |
|
-
Take a measurement of the drawing (width or length)
-
Take this same measurement of the specimen
-
Remember to convert units if needed to
-
Place your values into the equation
-
Magnification = length of drawing / length of actual specimen
You can also calculate the length of the specimen if this is unknown: length of the drawing / magnification.
Conversion of units: 1 centimeter = 10-2 meters 1 millimeter = 10-3 meters 1 micrometer = 10-6 meters 1 nanometer = 10-9 meters |
| |
2.1.6 Explain the importance of the surface area to volume ratio as a factor limiting cell size. |
|
Many reactions occur within the cell. Substances need to be taken into the cell to fuel these reactions and the wast products of the reactions need to be removed. When the cell increases in size so does its chemical activity. This means that more substances need to be taken in and more need to be removed. The surface area of the cell is vital for this. Surface area affects the rate at which particles can enter and exit the cell (The amount of substances that it takes up from the environment and excretes into the environment), whereas the volume affects the rate at which material are made or used within the cell, hence the chemical activity per unit of time.
As the volume of the cell increases so does the surface area however not to the same extent. When the cell gets bigger its surface area to volume ratio gets smaller. To illustrate this we can use three different cubes. The first cube has a side of 1 cm, the second 3 cm and the third 4 cm. If we calculate the surface area to volume ratio we get:
Cube 1 Surface area: 6 sides x 12 = 6 cm2 Volume: 13 = 1 cm3 Ratio = 6:1
Cube 2 Surface area: 6 sides x 32 = 54 cm2 Volume: 33 = 27 cm3 Ratio = 2:1
Cube 3 Surface area: 6 sides x 42 = 96 cm2 Volume : 43 = 64 cm3 Ratio = 1.5:1
As we can see the cube with the largest surface area and volume has the smallest surface area to volume ratio. If the surface area to volume ratio gets too small then substances won’t be able to enter the cell fast enough to fuel the reactions and wast products will start to accumulate within the cell as they will be produced faster than they can be excreted. In addition, cells will not be able to lose heat fast enough and so may overheat. Therefor the surface area to volume ratio is very important for a cell. |
| |
2.1.7 State that multicellular organisms show emergent properties. |
|
Multicellular organisms show emergent properties. For example: cells form tissues, tissues form organs, organs form organ systems and organ systems form multicellular organisms. The idea is that the whole is greater than the composition of its parts. For example your lungs are made of many cells. However, the cells by themselves aren’t much use. It is the many cells working as a unit that allow the lungs to perform their function. |
| |
2.1.8 Explain that cells in multicellular organisms differentiate to carry out specialized functions by expressing some of their genes but not others. |
|
Every cell in a multicellular organisms contains all the genes of that organism. However, the genes that are activated vary from cell to cell. The reason we have different types of cells in our body (the cells in your eyes are not the same as the ones that make up your hair) is because different genes are activated in different cells. For example, the gene that produces keratin will be active in hair and nail cells. Keratin is the protein which makes up hair and nails. Genes encode for proteins and the proteins affect the cell’s structure and function so that the cell can specialize. This means cells develop in different ways. This is called differentiation. Differentiation depends on gene expression which is regulated mostly during transcription. It is an advantage for multicellular organisms as cells can differentiate to be more efficient unlike unicellular organisms who have to carry out all of the functions within that one cell. |
| |
2.1.9 State that stem cells retain the capacity to divide and have the ability to differentiate along different pathways. |
|
Adults have stems cells in the tissues in their bodies that need to be frequently replaced such as the skin. Stem cells have the ability to produce a wide range of cells which means that they are pluripotent. They retain their ability to divide and produce many different cells by cell division and the process of differentiation. For example, one type of stem cells in the bone marrow produce a variety of red and white blood cells. |
| |
2.1.10 Outline one therapeutic use of stem cells. |
|
Bone marrow transplants are one of the many therapeutic uses of stem cells. Stem cells found in the bone marrow give rise to the red blood cells, white blood cells and platelets in the body. These stem cells can be used in bone marrow transplants to treat people who have certain types of cancer.
When a patient has cancer and is given high doses of chemotherapy, the chemotherapy kills the cancer cells but also the normal cells in the bone marrow. This means that the patient cannot produce blood cells. So before the patient is treated with chemotherapy, he or she can undergo a bone marrow harvest in which stem cells are removed from the bone marrow by using a needle which is inserted into the pelvis (hip bone). Alternatively, if stem cells cannot be used from the patient then they can be harvested from a matching donor. After the chemotherapy treatment the patient will have a bone marrow transplant in which the stem cells are transplanted back into the patient through a drip, usually via a vein in the chest or the arm. These transplanted stem cells will then find their way back to the bone marrow and start to produce healthy blood cells in the patient. Therefore the therapeutic use of stem cells in bone marrow transplants is very important as it allows some patients with cancer to undergo high chemotherapy treatment. Without this therapeutic use of stem cells, patients would only be able to take low doses of chemotherapy which could lower their chances of curing the disease.
|
| |
2.2.1 Draw and label a diagram of the ultrastructure of Escherichia coli (E. coli) as an example of a prokaryote. |
|
 |
| |
2.2.2 Annotate the diagram from 2.2.1 with the functions of each named structure. |
|
Cell wall: Protects the cell from the outside environment and maintains the shape of the cell. It also prevents the cell from bursting if internal pressure rises.
Plasma membrane: Semi-permeable membrane that controls the substances moving into and out of the cell. It contains integral and peripheral proteins. Substances pass through by either active or passive transport.
Cytoplasm: Contains many enzymes used to catalyze chemical reactions of metabolism and it also contains the DNA in a region called the nucleoid. Ribosomes are also found in the cytoplasm.
Pili: Help bacteria adhere to each other for the exchange of genetic material.
Flagella (singular flagellum): Made of a protein called flagellin. Helps bacteria move around by the use of a motor protein that spins the flagellum like a propeller.
Ribosomes: They are the site of protein synthesis. Contributes to protein synthesis by translating messenger RNA.
Nucleoid: Region containing naked DNA which stores the hereditary material (genetic information) that controls the cell and will be passed on to daughter cells. |
| |
2.2.3 Identify structures from 2.2.1 in electron micrographs of E. coli. |
|
 |
| |
2.2.4 State that prokaryotic cells divide by binary fission. |
|
Prokaryotic cells divide by binary fission. Binary fission is a method of asexual reproduction involving the splitting of the parent organism into two separate organisms. |
| |
2.3.1 Draw and label a diagram of the ultrastructure of a liver cell as an example of an animal cell. |
|

|
| |
2.3.2 Annotate the diagram from 2.3.1 with the functions of each named structure. |
|
Ribosomes: Found either floating free in the cytoplasm or attached to the surface of the rough endoplasmic reticulum and in mitochondria and chloroplast. Ribosomes are the site of protein synthesis as they translate messenger RNA to produce proteins. Rough endoplasmic reticulum: Can modify proteins to alter their function and/or destination. Synthesizes proteins to be excreted from the cell. Lysosome: Contains many digestive enzymes to hydrolyze macromolecules such as proteins and lipids into their monomers. Golgi apparatus: Receives proteins from the rough endoplasmic reticulum and may further modify them. It also packages proteins before the protein is sent to it’s final destination which may be intracellular or extracellular. Mitochondrion: Is responsible for aerobic respiration. Converts chemical energy into ATP using oxygen. Nucleus: Contains the chromosomes and therefore the hereditary material. It is responsible for controlling the cell. |
| |
2.3.3 Identify structures from 2.3.1 in electron micrographs of liver cells. |
|
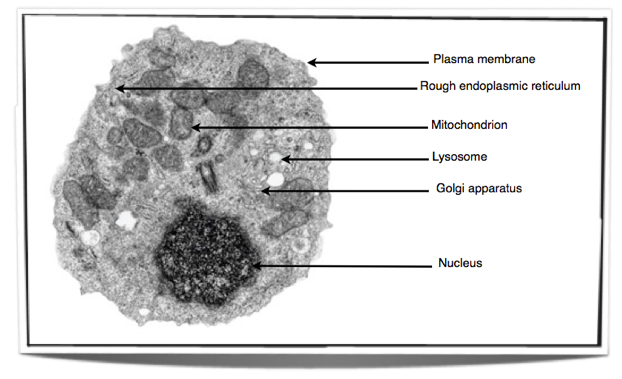
|
| |
2.3.4 Compare prokaryotic and eukaryotic cells. |
|
Prokaryotic cells have naked DNA which is found in the cytoplasm in a region named the nucleoid. On the other hand, eukaryotes have chromosomes that are made up of DNA and protein. These chromosomes are found in the nucleus enclosed in a nuclear envelope. Prokaryotes do not have any mitochondria whereas eukaryotes do. Prokaryotes have small ribosomes (70S) compared to eukaryotes which have large ribosomes (80S). In prokaryotes there are either no or very few organelles bounded by a single membrane in comparison to eukaryotes which have many of them including the Golgi apparatus and the endoplasmic reticulum. |
| |
2.3.5 State three differences between plant and animal cells. |
|
Animal cells only have a plasma membrane and no cell wall. Whereas plant cells have a plasma membrane and a cell wall. Animal cells do not have chloroplasts whereas plant cells do for the process of photosynthesis. Animal cells store glycogen as their carbohydrate resource whereas plants store starch. Animal cells do not usually contain any vacuoles and if present they are small or temporary. On the other hand plants have a large vacuole that is always present. Animal cells can change shape due to the lack of a cell wall and are usually rounded whereas plant cells have a fixed shape kept by the presence of the cell wall. |
| |
2.3.6 Outline two roles of extracellular components. |
|
The plant cell wall gives the cell a lot of strength and prevents it from bursting under high pressure as it is made up of cellulose arranged in groups called microfibrils. It gives the cell its shape, prevents excessive water up take by osmosis and is the reason why the whole plant can hold itself up against gravity. The animal cell contains glycoproteins in their extracellular matrix which are involved in the support, movement and adhesion of the cell. |
| |
2.4.1 Draw and label a diagram to show the structure of membranes. |
|

 |
| |
2.4.2 Explain how the hydrophobic and hydrophilic properties of phospholipids help to maintain the structure of cell membranes. |
|
Phospholipid molecules make up the cell membrane and are hydrophilic (attracted to water) as well as hydrophobic (not attracted to water but are attracted to other hydrophobic tails). They have a hydrophilic phosphate head and two hydrophobic hydrocarbon tails. Cell membranes are made up of a double layer of these phospholipid molecules. This is because in water the hydrophilic heads will face the water while the hydrophobic tails will be in the center because they face away from the water. The phospholipid bilayer makes the membrane very stable but also allows flexibility. The phospholipid in the membrane are in a fluid state which allows the cell to change it’s shape easily. |
| |
2.4.3 List the functions of membrane proteins. |
|
Membrane proteins can act as hormone binding sites, electron carriers, pumps for active transport, channels for passive transport and also enzymes. In addition they can be used for cell to cell communication as well as cell adhesion. |
| |
2.4.4 Define diffusion and osmosis. |
|
Diffusion is the passive movement of particles from a region of high concentration to a region of low concentration. Osmosis is the passive movement of water molecules, across a partially permeable membrane, from a region of lower solute concentration to a region of higher solute concentration. |
| |
2.4.5 Explain passive transport across membranes by simple diffusion and facilitated diffusion. |
|
Membranes are semi-permeable which means that they allow certain molecules through but not others. The molecules can move in and out through passive transport which is a method that does not require any input of outside energy. It can either be done by simple diffusion or facilitated diffusion. Molecules will go from a region of high concentration to a region of low concentration as they move randomly and eventually become evenly distributed within the system if they are permeable to the membrane. Simple diffusion involves the diffusion of molecules through the phospholipid bilayer while facilitated diffusion involves the use of channel proteins embedded in the membrane. The cell membrane is hydrophobic inside so hydrophobic (lipid soluble) molecules will pass through by simple diffusion whereas hydrophilic molecules and charged particles will use facilitated diffusion. Water moves through by osmosis which is also by passive transport. Osmosis involves the movement of water molecules from a region of low solute concentration, to a region of high solute concentration. So if the solute concentration is higher inside the cell than outside the cell, water will move in and vice versa. |
| |
2.4.6 Explain the role of protein pumps and ATP in active transport across membranes. |
|
Active transport involves the movement of substances through the membrane using energy from ATP. The advantage of active transport is that substances can be moved against the concentration gradient, meaning from a region of low concentration to a region of high concentration. This is possible because the cell membrane has protein pumps embedded it which are used in active transport to move substances across by using ATP. Each protein pump only transports certain substances so the cell can control what comes in and what goes out. |
| |
2.4.7 Explain how vesicles are used to transport materials within a cell between the rough endoplasmic reticulum, Golgi apparatus and the cell membrane. |
|
After proteins have been synthesized by ribosomes they are transported to the rough endoplasmic reticulum where they can be modified. Vesicles carrying the protein then bud off the rough endoplasmic reticulum and are transported to the Golgi apparatus to be further modified. After this the vesicles carrying the protein bud off the Golgi apparatus and carry the protein to the plasma membrane. Here the vesicles fuse with the membrane expelling their content (the modified proteins) outside the cell. The membrane then goes back to its original state. This is a process called exocytosis. Endocytosis is a similar process which involves the pulling of the plasma membrane inwards so that the pinching off of a vesicle from the plasma membrane occurs and then this vesicle can carry its content anywhere in the cell. |
| |
2.4.8 Describe how the fluidity of the membrane allows it to change shape, break and re-form during endocytosis and exocytosis. |
|
The phospholipids in the cell membrane are not solid but are in a fluid state allowing the membrane to change its shape and also vesicles to fuse with it. This means substances can enter the cell via endocytosis and exit the cell via exocytosis. The membrane then returns to its original state. In exocytosis the vesicles fuse with the membrane expelling their content outside the cell. The membrane then goes back to its original state. Endocytosis is a similar process which involves the pulling of the plasma membrane inwards so that a vesicle is pinched off it and then this vesicle can carry its content anywhere in the cell. |
| |
2.5.1 Outline the stages in the cell cycle, including interphase, (G1, S, G2), mitosis and cytokinesis. |
|
The first stage of cell division is interphase which is divided into 3 phases; G1, S and G2. The cell cycle starts with G1 (Gap phase 1) during which the cell grows larger. This is followed by phase S (synthesis) during which the genome is replicated. Finally, G2 (gap phase 2) is the second growth phase which separates the newly replicated genome and marks the end of interphase. The fourth stage is mitosis which is divided into prophase, metaphase, anaphase and telophase. During mitosis the spindle fibers attach to the chromosomes and pull sister chromatids apart. This stage separates the two daughter genomes. Finally, cytokinesis is the last stage during which the cytoplasm divides to create two daughter cells. In animal cells the cell is pinched in two while plant cells form a plate between the dividing cells. |
| |
2.5.2 State that tumors (cancers) are the result of uncontrolled cell division and that these can occur in any organ or tissue. |
|
Tumors are formed when cell division goes wrong and is no longer controlled. This can happen in any organ or tissue. |
| |
2.5.3 State that interphase is an active period in the life of a cell when many metabolic reactions occur, including protein synthesis, DNA replication and an increase in the number of mitochondria and/or chloroplast. |
|
Interphase is an active period in the life of a cell during which many metabolic reactions occur such as protein synthesis, DNA replication and an increase in the number of mitochondria and/or chloroplast. |
| |
2.5.4 Describe the events that occur in the four phases of mitosis (prophase, metaphase, anaphase, telophase). |
|
During prophase the spindle microtubules grow and extend from each pole to the equator. Also chromosomes super coil and become short and bulky and the nuclear envelope breaks down. During metaphase the chromatids move to the equator and the spindle microtubules from each pole attach to each centromere on opposite sides. During anaphase the spindle microtubules pull the sister chromatids apart splitting the centromeres. This splits the sister chromatids into chromosomes. Each identical chromosome is pulled to opposite poles. During telophase the spindle microtubules break down and the chromosomes uncoil and so are no longer individually visible. Also the nuclear membrane reforms. The cell then divides by cytokinesis to form two daughter cells with identical genetic nuclei. |
| |
2.5.5 Explain how mitosis produces two genetically identical nuclei. |
|
Mitosis is divided into four stages; prophase, metaphase, anaphase and telophase. During prophase, the chromosomes become visible under a light microscope as they super coil and therefore they get shorter and more bulky. The nuclear envelope disintegrates and the spindle microtubules grow and extend from each pole to the equator. At metaphase the chromatids move to the equator. The sister chromatids are two DNA molecules formed by DNA replication and are therefore identical. These sister chromatids are then separated in anaphase as the spindle microtubules attaches to centromere and pulls the sister chromatids to opposite poles. As the sister chromatids separate they are called chromosomes. This means that each pole has the same chromosomes (same genetic material). Finally the microtubules break down, the chromosomes uncoil and the nuclear membrane reforms. The cell then divides into two daughter cells with genetically identical nuclei. |
| |
2.5.6 State that growth, embryonic development, tissue repair and asexual reproduction involve mitosis. |
|
Growth, embryonic development, tissue repair and asexual reproduction involve mitosis |
| |
3.1.1 State that the most frequently occurring chemical elements in living things are carbon, hydrogen, oxygen and nitrogen. |
|
Carbon, hydrogen, oxygen and nitrogen are the most frequently occurring chemical elements in living things. |
| |
3.1.2 State that a variety of other elements are needed by living organisms, including sulfur, calcium, phosphorus, iron and sodium. |
|
A variety of other elements are needed by living organisms, including sulfur, calcium, phosphorus, iron and sodium. |
| |
3.1.3 State one role for each of the elements mentioned in 3.1.2. |
|
Sulfur: Needed for the synthesis of two amino acids. Calcium: Acts as a messenger by binding to calmodulin and a few other proteins which regulate transcription and other processes in the cell. Phosphorus: Is part of DNA molecules and is also part of the phosphate groups in ATP. Iron: Is needed for the synthesis of cytochromes which are proteins used during electron transport for aerobic cell respiration. Sodium: When it enters the cytoplasm, it raises the solute concentration which causes water to enter by osmosis. |
| |
3.1.4 Draw and label a diagram showing the structure of water molecules to show their polarity and hydrogen bond formation. |
|

|
| |
3.1.5 Outline the thermal, cohesive and solvent properties of water. |
|
Thermal properties of water include heat capacity, boiling and freezing points and the cooling effect of evaporation. Water has a large heat capacity which means that a considerable amount of energy is needed to increase it’s temperature. This is due to the strength of the hydrogen bonds which are not easily broken. This is why the temperature of water tends to remain relatively stable. It is beneficial for aquatic animals as they use water as a habitat. Water has a high boiling and freezing point. It boils at 100 C because the strong hydrogen bonds. All these hydrogen bonds between the water molecules need to break for the liquid to change to gas. Water becomes less dense as it gets closer to the freezing point and so ice always forms on the surface first. The high boiling point of water is vital for life on earth as if water boiled at a lower temperature the water in living organisms would start to boil and therefore these organisms would not survive. The fact that water becomes less dense as it freezes is beneficial to organisms as ice will always form at the surface of lakes or seas and by doing so it insulates the water underneath, maintaining a possible habitat for organisms to live in. Water can evaporate at temperatures below the boiling point. Hydrogen bonds need to break for this to occur. Cohesion is the effect of hydrogen bonds holding the water molecules together. Water moves up plants because of cohesion. Long columns of water can be sucked up from roots to leaves without the columns breaking. The hydrogen bonds keep the water molecules sticking to each other. The solvent properties of water mean that many different substances can dissolve in it because of its polarity. |
| |
3.1.6 Explain the relationship between the properties of water and its uses in living organisms as a coolant, medium for metabolic reactions and transport medium. |
|
Water can evaporate at temperatures below the boiling point. Hydrogen bonds need to break for this to occur. The evaporation of water cools body surfaces (sweat) and plant leaves (transpiration) by using the energy from liquid water to break the hydrogen bonds. The solvent properties of water mean that many different substances can dissolve in it because of its polarity. This allows substances to be carried in the blood and sap of plants as they dissolve in water. It also makes water a good medium for metabolic reactions. |
| |
3.2.1 Distinguish between organic and inorganic compounds. |
|
Organic compounds are compounds that are found in living organisms and contain carbon. Inorganic compounds are the ones that don’t contain carbon. Although, there are a few compounds found in living organisms which also contain carbon but are considered as inorganic compounds. These include carbon dioxide, carbonates and hydrogen carbonates. |
| |
3.2.2 Identify amino acids, glucose, ribose and fatty acids from diagrams showing their structure. |
|


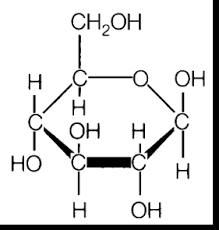
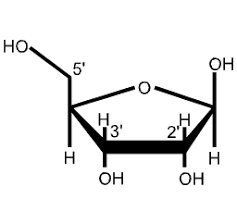
|
| |
3.2.3 List three examples of each monosaccharides, disaccharides and polysaccharides. |
|
- Glucose, galactose and fructose are all monosaccharides.
- Maltose, lactose and sucrose are all disaccharides.
- Starch, glycogen and cellulose are all polysaccharides.
|
| |
3.2.4 State one function of glucose, lactose and glycogen in animals, and of fructose, sucrose and cellulose in plants. |
|
In animals, glucose is used as an energy source for the body and lactose is the sugar found in milk which provides energy to new borns until they are weaned. Finally, glycogen is used as an energy source (short term only) and is stored in muscles and the liver. In plants, fructose is what makes fruits taste sweet which attracts animals and these then eat the fruits and disperse the seeds found in the fruits. Sucrose is used as an energy source for the plant whereas cellulose fibers is what makes the plant cell wall strong. |
| |
3.2.5 Outline the role of condensation and hydrolysis in the relationships between monosaccharides, disaccharides and polysaccharides; between fatty acids, glycerol and triglycerides; and between amino acids and polypeptides. |
|
In a condensation reaction the bond between an oxygen and a hydrogen is broken. The hydrogen will move to an OH group and attach to the oxygen. This causes the bond between this oxygen and the carbon broken. This leaves one molecule with an oxygen with one lone electron and another molecule with a carbon with one lone electron. The 2 electrons join and form a new covalent bond. |
| |
3.2.6 State three functions of lipids. |
|
- Lipids can be used for energy storage in the form of fat in humans and oil in plants.
- Lipids can be used as heat insulation as fat under the skin reduces heat loss.
- Lipids allow buoyancy as they are less dense than water and so animals can float in water.
|
| |
3.2.7 Compare the use of carbohydrates and lipids in energy storage. |
|
Carbohydrates and lipids can both be used as energy storage however carbohydrates are usually used for short term storage whereas lipids are used for long term storage. Carbohydrates are soluble in water unlike lipids. This makes carbohydrates easy to transport around the body (from and to the store). Also, carbohydrates are a lot easier and more rapidly digested so their energy is useful if the body requires energy fast. As for lipids, they are insoluble which makes them more difficult to transport however because they are insoluble, lipids do not have an effect on osmosis which prevents problems within the cells in the body. They also contain more energy per gram than carbohydrates which makes lipids a lighter store compared to a store of carbohydrates equivalent in energy. |
| |
3.3.1 Outline DNA nucleotide structure in terms of sugar (deoxyribose), base and phosphate. |
|
A nucleotide is made of the sugar deoxyribose, a base (which can be either adenine, guanine, cytosine or thymine) and a phosphate group. Below is a representation of a nucleotide. |
| |
3.3.2 State the names of the four bases in DNA. |
|
adenine, Guanine, Cytosine and Thymine. |
| |
3.3.3 Outline how DNA nucleotides are linked together by covalent bonds into a single strand |
|
A covalent bond forms between the sugar of one nucleotide and the phosphate group of another nucleotide. |
| |
3.3.4 Explain how a DNA double helix is formed using complementary base pairing and hydrogen bonds. |
|
DNA is made up of two nucleotide strands. The nucleotides are connected together by covalent bonds within each strand. The sugar of one nucleotide forms a covalent bond with the phosphate group of another. The two strands themselves are connected by hydrogen bonds. The hydrogen bonds are found between the bases of the two strands of nucleotides. Adenine forms hydrogen bonds with thymine whereas guanine forms hydrogen bonds with cytosine. This is called complementary base pairing. Below is a digram showing the molecular structure and bonds within DNA. |
| |
3.3.5 Draw and label a simple diagram of the molecular structure of DNA. |
|
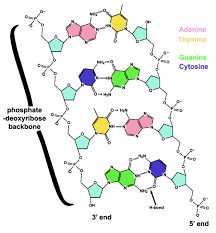
|
| |
3.4.1 Explain DNA replication in terms of unwinding the double helix and separation of the strands by helicase, followed by formation of the new complementary strands by DNA polymerase. |
|
DNA replication is semi-conservative as both of the DNA molecules produced are formed from an old strand and a new one. The first stage of DNA replication involves the unwinding of the double strand of DNA (DNA double helix) and separating them by breaking the hydrogen bonds between the bases. This is done by the enzyme helicase. Each separated strand now is a template for the new strands. There are many free nucleotides around the replication fork which then bond to the template strands. The free nucleotides form hydrogen bonds with their complimentary base pairs on the template strand. Adenine will pair up with thymine and guanine will pair up with cytosine. DNA polymerase is the enzyme responsible for this. The new DNA strands then rewind to form a double helix. The replication process has produced a new DNA molecule which is identical to the initial one. |
| |
3.4.2 Explain the significance of complementary base pairing in the conservation of the base sequence of DNA. |
|
Complementary base pairing is very important in the conservation of the base sequence of DNA. This is because adenine always pairs up with thymine and guanine always pairs up with cytosine. As DNA replication is semi-conservative (one old strand an d one new strand make up the new DNA molecules), this complementary base pairing allows the two DNA molecules to be identical to each other as they have the same base sequence. The new strands formed are complementary to their template strands but also identical to the other template. Therefore, complementary base pairing has a big role in the conservation of the base sequence of DNA. |
| |
3.4.3 State that DNA replication is semi-conservative. |
|
DNA replication is semi-conservative. |
| |
3.5.1 Compare the structure of RNA and DNA. |
|
DNA and RNA both consist of nucleotides which contain a sugar, a base and a phosphate group. However there are a few differences. Firstly, DNA is composed of a double strand forming a helix whereas RNA is only composed of one strand. Also the sugar in DNA is deoxyribose whereas in RNA it is ribose. Finally, both DNA and RNA have the bases adenine, guanine and cytosine. However DNA also contains thymine which is replaced by uracil in RNA. |
| |
3.5.2 Outline DNA transcription in terms of the formation of an RNA strand complementary to the DNA strand by RNA polymerase. |
|
DNA transcription is the formation of an RNA strand which is complementary to the DNA strand. The first stage of transcription is the uncoiling of the DNA double helix. Then, the free RNA nucleotides start to form an RNA strand by using one of the DNA strands as a template. This is done through complementary base pairing, however in the RNA chain, the base thymine is replaced by uracil. RNA polymerase is the enzyme involved in the formation of the RNA strand and the uncoiling of the double helix. The RNA strand then elongates and then separates from the DNA template. The DNA strands then reform a double helix. The strand of RNA formed is called messenger RNA. |
| |
3.5.3 Describe the genetic code in terms of codons composed of triplets of bases. |
|
A triplet of bases (3 bases) forms a codon. Each codon codes for a particular amino acid. Amino acids in turn link to form proteins. Therefore DNA and RNA regulate protein synthesis. The genetic code is the codons within DNA and RNA, composed of triplets of bases which eventually lead to protein synthesis. |
| |
3.5.4 Explain the process of translation, leading to polypeptide formation. |
|
Translation is the process through which proteins are synthesized. It uses ribosomes, messenger RNA which is composed of codons and transfer RNA which has a triplet of bases called the anticodon. The first stage of translation is the binding of messenger RNA to the small subunit of the ribosome. The transfer RNA’s have a specific amino acid attached to them which corresponds to their anticodons. A transfer RNA molecule will bind to the ribosome however it’s anticodon must match the codon on the messenger RNA. This is done through complementary base pairing. These two form a hydrogen bond together. Another transfer RNA molecule then bonds. Two transfer RNA molecules can bind at once. Then the two amino acids on the two transfer RNA molecules form a peptide bond. The first transfer RNA then detaches from the ribosome and the second one takes it’s place.The ribosome moves along the messenger RNA to the next codon so that another transfer RNA can bind. Again, a peptide bond is formed between the amino acids and this process continues. This forms a polypeptide chain and is the basis of protein synthesis. |
| |
3.5.5 Discuss the relationship between one gene and one polypeptide. |
|
A polypeptide is formed by amino acids liking together through peptide bonds. There are 20 different amino acids so a wide range of polypeptides are possible. Genes store the information required for making polypeptides. The information is stored in a coded form by the use of triplets of bases which form codons. The sequence of bases in a gene codes for the sequence of amino acids in a polypeptide. The information in the genes is decoded during transcription and translation leading to protein synthesis. |
| |
3.6.1 Define enzyme and active site. |
|
Enzymes: Globular proteins which act as catalysts of chemical reactions. Active site: Region on the surface of an enzyme to which substrates bind and which catalyses a chemical reaction involving the substrates. |
| |
3.6.2 Explain enzyme–substrate specificity. |
|
The active site of an enzyme is very specific to its substrates as it has a very precise shape. This results in enzymes being able to catalyze only certain reactions as only a small number of substrates fit in the active site. This is called enzyme-substrate specificity. For a substrate to bind to the active site of an enzyme it must fit in the active site and be chemically attracted to it. This makes the enzyme very specific to it’s substrate. The enzyme-substrate complex can be compared to a lock and key, where the enzyme is the lock and the substrate is the key. |
| |
3.6.3 Explain the effects of temperature, pH and substrate concentration on enzyme activity. |
|
Enzyme activity increases with an increase in temperature and usually doubles with every 10 degrees rise. This is due to the molecules moving faster and colliding more often together. However at a certain point the temperature gets to high and the enzymes denature and stop functioning. This is due to the heat causing vibrations within the enzyme destroying its structure by breaking the bonds in the enzyme. Enzymes usually have an optimum pH at which they work most efficiently. As the pH diverges from the optimum, enzyme activity decreases. Both acid and alkali environments can denature enzymes. Enzyme activity increases with an increase in substrate concentration as there are more random collisions between the substrate and the active site. However, at some point, all the active sites are taken up and so increasing the substrate concentration will have no more effect on enzyme activity. As long as there are active sites available, an increase in substrate concentration will lead to an increase in enzyme activity. |
| |
3.6.4 Define denaturation. |
|
Denaturation is changing the structure of an enzyme (or other protein) so it can no longer carry out its function. |
| |
3.6.5 Explain the use of lactase in the production of lactose-free milk. |
|
Lactose is the sugar found in milk. It can be broken down by the enzyme lactase into glucose and galactose. However some people lack this enzyme and so cannot break down lactose leading to lactose intolerance. Lactose intolerant people need to drink milk that has been lactose reduced. Lactose-free milk can be made in two ways. The first involves adding the enzyme lactase to the milk so that the milk contains the enzyme. The second way involves immobilizing the enzyme on a surface or in beads of a porous material. The milk is then allowed to flow past the beads or surface with the immobilized lactase. This method avoids having lactase in the milk. |
| |
3.7.1 Define cell respiration |
|
Cell respiration is the controlled release of energy from organic compounds in cells to form ATP. |
| |
3.7.2 State that, in cell respiration, glucose in the cytoplasm is broken down by glycolysis into pyruvate, with a small yield of ATP. |
|
In cell respiration, glucose in the cytoplasm is broken down by glycolysis into pyruvate with a small yield of ATP. |
| |
3.7.3 Explain that, during anaerobic cell respiration, pyruvate can be converted in the cytoplasm into lactate, or ethanol and carbon dioxide, with no further yield of ATP. |
|
In anaerobic cell respiration the pyruvate stays in the cytoplasm and in humans is converted into lactate which is the removed from the cell. In yeast the pyruvate is converted into carbon dioxide and ethanol. In either case, no ATP is produced. |
| |
3.7.4 Explain that, during aerobic cell respiration, pyruvate can be broken down in the mitochondrion into carbon dioxide and water with a large yield of ATP. |
|
If oxygen is available, the pyruvate is taken up into the mitochondria and is broken down into carbon dioxide and water. A large amount of ATP is released during this process. |
| |
3.8.1 State that photosynthesis involves the conversion of light energy into chemical energy. |
|
Photosynthesis involves the conversion of light energy into chemical energy. |
| |
3.8.2 State that light from the Sun is composed of a range of wavelengths (colours). |
|
The light from the sun is composed of a range of wavelengths (colours). |
| |
3.8.3 State that chlorophyll is the main photosynthetic pigment. |
|
Chlorophyll is the main photosynthetic pigment. |
| |
3.8.4 Outline the differences in absorption of red, blue and green light by chlorophyll. |
|
Chlorophyll can absorb red and blue light more than green. Chlorophyll cannot absorb green light and so instead reflects it making leaves look green. |
| |
3.8.5 State that light energy is used to produce ATP, and to split water molecules (photolysis) to form oxygen and hydrogen. |
|
Light energy is used to produced ATP and to split water molecules (photolysis) to form oxygen and hydrogen. |
| |
3.8.6 State that ATP and hydrogen (derived from the photolysis of water) are used to fix carbon dioxide to make organic molecules. |
|
ATP and hydrogen derived from photolysis of water are used to fix carbon dioxide to make organic molecules. |
| |
3.8.7 Explain that the rate of photosynthesis can be measured directly by the production of oxygen or the uptake of carbon dioxide, or indirectly by an increase in biomass. |
|
Photosynthesis can be measured in many ways as it involves the production of oxygen, the uptake of carbon dioxide and an increase in biomass. For example, aquatic plants release oxygen bubbles during photosynthesis and so these can be collected and measured. The uptake of carbon dioxide is more difficult to measure so it is usually done indirectly. When carbon dioxide is absorbed from water the pH of the water rises and so this can be measured with pH indicators or pH meters. Finally, photosynthesis can be measured through an increase in biomass. If batches of plants are harvested at a series of times and the biomass of these batches is calculated, the rate increase in biomass gives an indirect measure of the rate of photosynthesis in the plants. |
| |
3.8.8 Outline the effects of temperature, light intensity and carbon dioxide concentration on the rate of photosynthesis. |
|
As temperature increases, the rate of photosynthesis increases more and more steeply until the optimum temperature is reached. If temperature keeps increasing above the optimum temperature then photosynthesis starts to decrease very rapidly. As light intensity increases so does photosynthesis until a certain point. At a high light intensities photosynthesis reaches a plateau and so does not increase any more. At low and medium light intensity the rate of photosynthesis is directly proportional to the light intensity. As the carbon dioxide concentration increases so does the rate of photosynthesis. There is no photosynthesis at very low levels of carbon dioxide and at high levels the rate reaches a plateau. |
| |
4.1.1 State that eukaryote chromosomes are made of DNA and proteins. |
|
Eukaryote chromosomes are made of DNA and proteins. |
| |
4.1.2 Define gene, allele and genome. |
|
Gene: a heritable factor that controls a specific characteristic. Allele: one specific form of a gene, differing from other alleles by one or a few bases only and occupying the same gene locus as other alleles of the gene. Genome: the whole of the genetic information of an organism. |
| |
4.1.3 Define gene mutation. |
|
Gene mutation: a change to the base sequence of a gene. |
| |
4.1.4 Explain the consequence of a base substitution mutation in relation to the processes of transcription and translation, using the example of sickle-cell anemia. |
|
Sickle cell anaemia is a genetic disease that affects red blood cells in the body. It is due to a mutation on the Hb gene which codes for a polypeptide of 146 amino acids which is part of haemoglobin (haemoglobin is an important protein component in red blood cells). In sickle cell anaemia the codon GAG found in the normal Hb gene is mutated to GTG. This is called a base substitution mutation as adenine (A) is replaced by thymine (T). This means that when the mutated gene is transcribed, a codon in the messenger RNA will be different. Instead of the normal codon GAG, the messenger RNA will contain the codon GUG. This in turn will result in a mistake during translation. In a healthy individual the codon GAG on the messenger RNA matches with the anticodon CUC on the transfer RNA carrying the amino acid glutamic acid. However, if the mutated gene is present then GUG on the messenger RNA matches with the anticodon CAC on the transfer RNA which carries the amino acid valine. So the base substitution mutation has caused glutamic acid to be replaced by valine on the sixth position on the polypeptide. This results in haemoglobin S being present in red blood cells instead of the normal haemoglobin A. This has an effect on the phenotype as instead of normal donut shaped red blood cells being produced some of the red blood cells will be sickle shaped. As a result these sickle shaped red blood cells cannot carry oxygen as efficiently as normal red blood cells would. However, there is an advantage to sickle cell anemia. The sickle cell red blood cells give resistance to malaria and so the allele Hbs on the Hb gene which causes sickle cell anemia is quite common in parts of the world where malaria is found as it provides an advantage over the disease. Summary of important steps: Normal Gene Mutated gene Codon GAG GTG Transcription GAG on mRNA GUG on mRNA Translation Anticodon CUC and amino acid glutamic acid on tRNA. Anticodon CAC and amino acid valine on tRNA. Haemoglobin HbA HbS Phenotype Normal donut shaped red blood cells. Sickle cell shaped red blood cells. Effects Carry oxygen efficiently but are affected by malaria. Do not carry oxygen efficiently but give resistance to malaria. |
| |
4.2.1 State that meiosis is a reduction division of a diploid nucleus to form haploid nuclei. |
|
Meiosis is a reduction division of a diploid nucleus to form haploid nuclei. |
| |
4.2.2 Define homologous chromosomes. |
|
Homologous chromosomes: chromosomes with the same genes as each other, in the same sequence but do not necessarily have the same allele of those genes. |
| |
4.2.3 Outline the process of meiosis, including pairing of homologous chromosomes and crossing over, followed by two divisions, which results in four haploid cells. |
|
Meiosis involves two divisions. Meiotic cells have an interphase stage before the start of meiosis I which is similar to mitosis. It includes G1, S and G2 phases. (See notes on mitosis) After meiosis I there is another brief interphase stage which is followed by meiosis II.Meiosis I The first stage of meiosis I is prophase I. In prophase I the chromosomes pair up so that the chromosomes in each pair are homologous. Once the homologous chromosomes are paired up, crossing over occurs. Crossing over is the exchange of genetic material between non-sister chromatids. The nuclear membrane also starts to break down and the spindle microtubules stretch out from each pole to the equator. The second stage is metaphase I. Here the paired up homologous chromosomes line up at the equator and the spindle fibbers attach to the chromosomes in a way that ensures that for each homologous pair, one chromosome moved to one pole and the other moves to the opposite pole. The third stage is anaphase I. This is the stage where the homologous chromosomes are separated and pulled to opposite poles. This halves the chromosome number however each chromosome is still composed of two sister chromatids. The cell membrane starts to prepare for its separation at the equator to form two cells. The fourth stage is telophase I. Here each chromosome from the homologous pair are found at opposite poles and the nuclear membrane reforms around each daughter nucleus. The membrane then divides through citokinesis. There is a brief interphase stage before the start of meiosis II. This stage does not include the S phase. Meiosis II The first stage of meiosis II is prophase II. Here the cell has divided into two daughter haploid cells however the process does not end here as these two cells immediately start to divide again. The spindle microtubules stretch out from each pole again and the nuclear membrane breaks down as in prophase I. The second stage is metaphase II. Here the chromosomes in each cell line up at the equator and the spindle microtubules attach to the centromere of each chromosome. The third stage is anaphase II. Here the centromere devised as a result of the spindle microtubules pulling each sister chromatid to opposite poles in both cells. Each sister chromatid then becomes a chromosome. The fourth stage is telophase II. Here the nuclear membrane reforms around the four sets of daughter chromosomes. Cytokinesis then follows to divide the cytoplasm of the two cells and so the result is four daughter cells each with a haploid set of chromosomes.
|
| |
4.2.4 Explain that non-disjunction can lead to changes in chromosome number, illustrated by reference to Down syndrome (trisomy 21). |
|

A number of problems can arise during meiosis. A common problem is non-disjunction. This is when the chromosomes do not separate properly during meiosis, either in meiosis I (in anaphase I) or meiosis II (in anaphase II). This leads the production of gametes that either have a chromosome too many or too few. Gametes with a missing chromosome usually die quite fast however gametes with an extra chromosome can survive. When a zygote is formed from the fertilization of these gametes with an extra chromosome, three chromosomes of one type are present instead of two. An example of this is Down syndrome. Down syndrome is a disease in which the chromosomes failed to separate properly during meiosis leading to three chromosomes of type 21 instead of two. A person with the condition therefore has a total of 47 chromosomes instead of 46. The non-disjunction can take place either in the formation of the egg or the sperm. Down syndrome leads to many complications and also the risk of having a child with the condition increases with age.
|
| |
4.2.5 State that, in karyotyping, chromosomes are arranged in pairs according to their size and structure. |
|
n karyotyping, chromosomes are arranged in pairs according to their size and structure. |
| |
4.2.6 State that karyotyping is performed using cells collected by chorionic villus sampling or amniocentesis, for pre-natal diagnosis of chromosome abnormalities. |
|
Karyotyping is performed using cells collected by chorionic villus sampling or amniocentesis, for pre-natal diagnosis of chromosome abnormalities. |
| |
4.2.7 Analyse a human karyotype to determine gender and whether non-disjunction has occurred. |
|
Karyotyping can be used to determine gender of a fetus and look for chromosome abnormalities such as non-disjunction. The gender can be deduced by looking at the sex chromosomes. Females will have two X chromosomes while males have one X and one Y. We can distinguish this on with karyotyping as the Y chromosome is smaller than the X. As for non-disjunctions we can see if a chromosome is missing or if their is an extra one by looking at the number of chromosomes. If There should only be two of each chromosome. Each 23 chromosomes should have a pair resulting in 46 chromosomes in total. For example, if we notice that there are three chromosomes 21 then we can conclude that a non-disjunction occurred. In this case, the non-disjunction results in Down’s syndrome. (trisomy 21) |
| |
4.3.1 Define genotype, phenotype, dominant allele, recessive allele, codominant alleles, locus, homozygous, heterozygous, carrier and test cross. |
|
Genotype: the alleles of an organism. Phenotype: the characteristics of an organism. Dominant allele: an allele that has the same effect on the phenotype whether it is present in the homozygous or heterozygous state. Recessive allele: an allele that only has an effect on the phenotype when present in the homozygous state. Codominant alleles: pairs of alleles that both affect the phenotype when present in a heterozygote. Locus: the particular position on homologous chromosomes of a gene. Homozygous: having two identical alleles of a gene. Heterozygous: having two different alleles of a gene. Carrier: an individual that has one copy of a recessive allele that causes a genetic disease in individuals that are homozygous for this allele. Test cross: testing a suspected heterozygote by crossing it with a known homozygous recessive. |
| |
4.3.2 Determine the genotypes and phenotypes of the offspring of a monohybrid cross using a Punnett grid. |
|

 |
| |
4.3.3 State that some genes have more than two alleles (multiple alleles). |
|
Some genes have more than two alleles (multiple alleles). |
| |
4.3.4 Describe ABO blood groups as an example of codominance and multiple alleles. |
|
The ABO blood group is a good example of codominance and multiple alleles. There are three allele that control the ABO blood groups. If there are more than two allele of a gene then they are called multiple allele. The allele IA corresponds to blood group A (genotype IAIA) and the allele IB corresponds to blood group B (genotype IBIB). Both of these are dominant and so if IA and IB are present together they form blood group AB (genotype IAIB). Both allele affect the phenotype since they are both codominant. Codominant allele are pairs of allele that both affect the phenotype when present together in a heterozygote. The allele i is recessive to both IA and IB so if you have the genotype IA i you will have blood group A and if you have the genotype IB i you will have blood group B. However if you have the genotype ii then you are homozygous for i and will be of blood group O. Below is a table to summaries which genotypes give which phenotypes. Phenotype Genotype A IAIA or IAi B IBIB or IBi AB IAIB O ii |
| |
4.3.5 Explain how the sex chromosomes control gender by referring to the inheritance of X and Y chromosomes in humans. |
|
There are two chromosomes which determine gender. These are called the sex chromosomes and there are two types, the X and the Y chromosome. Females have two X chromosomes whereas males have one X and one Y chromosome. The X chromosome is relatively large compared to the Y (which is much smaller) and contains many genes. The Y chromosome on the other hand only contains a few genes. The female always passes on to her offspring the X chromosome from the egg (female gamete). The male can pass on either the Y or the X chromosome from the sperm (male gamete). If the male passes on the X chromosome then the growing embryo will develop into a girls. If the male passes on the Y chromosome then the growing embryo will develop into a boy. Therefore gender depends on whether the sperm which fertilizes the egg is carrying an X or a Y chromosome. |
| |
4.3.6 State that some genes are present on the X chromosome and absent from the shorter Y chromosome in humans. |
|
Some genes are present on the X chromosome and absent from the shorter Y chromosome in humans. |
| |
4.3.7 Define sex linkage. |
|
Sex linkage: when the gene controlling the characteristic is located on the sex chromosome and so we associate the characteristic with gender. |
| |
4.3.8 Describe the inheritance of colour blindness and hemophilia as examples of sex linkage. |
|
Most of the time sex-linked genes are carried on the X chromosome. Since females have two X chromosomes they have two copies of the sex-linked gene whereas males only have one since they only have one X chromosome. Hemophilia and colour blindness are both examples of sex linkage. Hemophilia XH is the allele for normal blood clotting and is dominate over Xh which is recessive and causes hemophilia. If a mother is heterozygous she is a carrier of the disease but does not have hemophilia as the dominate allele is present. She can however pass the disease on to her offspring. Below is a punnett showing how a carrier mother and an unaffected father can pass the disease on to their offspring.
From our four possible outcomes we can see that a female child cannot get hemophilia but can be a carrier. This is because the father will always pass on the dominate allele (XH) on the X chromosome in females. Depending on whether the mother passes on the dominant or recessive allele will determine if the female child is a carrier or is unaffected by the hemophilia. If the child is a boy then the father has passed on the Y chromosome which does not contain the allele of the gene. So whether the child has the disease or is unaffected depends on which allele the mother had passed on. If she has passed on the recessive allele (Xh) then the male child will have hemophilia, however if she has passed on the dominate allele (XH) then the child will be unaffected. So there is a 50% chance of the child having hemophilia if it is male as half of the eggs produced by the mother will carry the recessive allele. The chance of a female offspring having hemophilia is 0% since the father always passes on the dominant allele on the X chromosome. Finally there is a 25% chance overall that the offspring will be affected. |
| |
4.3.9 State that a human female can be homozygous or heterozygous with respect to sex-linked genes. |
|
A human female can be homozygous or heterozygous with respect to sex-linked genes. |
| |
4.3.10 Explain that female carriers are heterozygous for X-linked recessive alleles. |
|
Female carriers for X-linked recessive alleles are always heterozygous since they require a dominant allele and a recessive allele to be carriers. They inherit the recessive allele from one parent and the dominate allele from the other. For example hemophilia is a sex-linked disease. If a carrier mother and an unaffected father have offspring then the unaffected father will always pass on his dominate allele to his female offspring. The carrier mother can either pass on the dominate or recessive allele. If she passes on the recessive allele to her female offspring than the female offspring will be a carrier as well. |
| |
4.3.11Predict the genotypic and phenotypic ratios of offspring of monohybrid crosses involving any of the above patterns of inheritance. |
|

Crossing between two heterozygous individuals gives a 3:1 ratio if one of the alleles is dominate and the other is recessive. |
| |
4.3.12 Deduce the genotypes and phenotypes of individuals in pedigree charts. |
|

- Squares represent males
- Circles represent females
- Shaded symbols represent affected individuals
- Unshaded symbols represent unaffected individuals.

- If most of the males in the pedigree are affected the disorder is X-linked.
- If it is a 50/50 ratio between men and women the disorder is autosomal.
- If the disorder is dominant, one of the parents must have the disorder.
- If the disorder is recessive than neither of the parents has to have the disorder as they can be heterozygous.
|
| |
4.4.1 Outline the use of polymerase chain reaction (PCR) to copy and amplify minute quantities of DNA. |
|
Polymerase chain reaction is used to copy and amplify minute quantities of DNA. It can be useful when only a small amount of DNA is available but a large amount is required to undergo testing. We can use DNA from blood, semen, tissues and so on from crime scenes for example. The PCR requires high temperature and a DNA polymerase enzyme from Thermus aquaticus (a bacterium that lives in hot springs). |
| |
4.4.2 State that, in gel electrophoresis, fragments of DNA move in an electric field and are separated according to their size. |
|
In gel electrophoresis, fragments of DNA move in an electrical field and are separated according to their size. |
| |
4.4.3 State that gel electrophoresis of DNA is used in DNA profiling. |
|
Gel electrophoresis of DNA is used in DNA profiling. |
| |
4.4.4 Describe the application of DNA profiling to determine paternity and also in forensic investigations. |
|
Organisms have short sequences of bases which are repeated many times. These are called satellite DNA. These repeated sequences vary in length from person to person. The DNA is copied using PCRand then cut up into small fragments using restriction enzymes. Gel electrophoresis separates fragmented pieces of DNA according to their size and charge. This gives a pattern of bands on a gel which is unlikely to be the same for two individuals. This is called DNA profiling. DNA profiling can be used to determine paternity and also in forensic investigations to get evidence to be used in a court case for example. |
| |
4.4.5 Analyse DNA profiles to draw conclusions about paternity or forensic investigations. |
|
For a suspect look for similarities between the DNA found at the crime scene and the suspect. For a paternity test, look for similarities between the child and the possible father. |
| |
4.4.6 Outline three outcomes of the sequencing of the complete human genome. |
|
- It is now easier to study how genes influence human development.
- It helps identify genetic diseases.
- It allows the production of new drugs based on DNA base sequences of genes or the structure of proteins coded for by these genes.
- It will give us more information on the origins, evolution and migration of humans.
|
| |
4.4.7 State that, when genes are transferred between species, the amino acid sequence of polypeptides translated from them is unchanged because the genetic code is universal. |
|
When genes are transferred between species, the amino acid sequence of polypeptides translated from them is unchanged because the genetic code is universal. |
| |
4.4.8 Outline a basic technique used for gene transfer involving plasmids, a host cell (bacterium, yeast or other cell), restriction enzymes (endonucleases) and DNA ligase. |
|
The human gene that codes for insulin can be inserted into a plasmid and then this plasmid can be inserted into a host cell such as a bacterium. The bacterium can then synthesis insulin which can be collected and used by diabetics. This is done as follows. The messenger RNA which codes for insulin is extracted from a human pancreatic cell which produces insulin. DNA copies are then made from this messenger RNA by using the enzyme reverse transcriptase and these DNA copies are then given extra guanine nucleotides to the end of the gene to create sticky ends. At the same time, a selected plasmid is cut using restriction enzymes which cut the DNA at specific base sequences. Then extra cytosine nucleotides are added to create sticky ends. Once we have both the plasmid and the gene ready, these are mixed together. The two will link by complementary base pairing (between cytosine and guanine) and then DNA ligase is used to make the sugar phosphate bonds. The plasmids with the human insulin gene (called recombinant plasmids) can then be mixed with host cells such as bacterium. The bacterium will take in the plasmid and start producing insulin which can then be collected and purified. |
| |
4.4.9 State two examples of the current uses of genetically modified crops or animals. |
|
- The transfer of a gene for factor IX which is a blood clotting factor, from humans to sheep so that this factor is produced in the sheep’s milk.
- The transfer of a gene that gives resistance to the herbicide glyphosate from bacterium to crops so that the crop plants can be sprayed with the herbicide and not be affected by it.
|
| |
4.4.10 Discuss the potential benefits and possible harmful effects of one example of genetic modification. |
|
It is quite common to see genetic modifications in crop plants. An example of this is the transfer of a gene that codes for a protein called Bt toxin from the bacterium Bacillus thuringiensis to maize crops. This is done because maize crops are often destroyed by insects that eat the corn and so by adding the Bt toxin gene this is prevented as the toxin kills the insects. However this is very controversial as even though it has many positive advantages, it can also have some harmful consequences. The table below summarizes the benefits and possible harmful effects of genetically modifying the maize crops. Benefits Harmful Effects Since there is less damage to the maize crops, there is a higher crop yield which can lessen food shortages. We are not sure of the consequences of humans and animals eating the modified crops. The bacterial DNA or the Bt toxin itself could be harmful to human as well as animal health. Since there is a higher crop yield, less land is needed to grow more crops. Instead the land can become an area for wild life conservation. Other insects which are not harmful to the crops could be killed. The maize pollen will contain the toxin and so if it is blown onto near by plants it can kill the insects feeding on these plants. There is a reduction in the use of pesticides which are expensive and may be harmful to the environment, wild life and farm workers. Cross pollination can occur which results in some wild plants being genetically modified as they will contain the Bt gene. These plants will have an advantage over others as they will be resistant to certain insects and so some plants may become endangered. This will have significant consequences on the population of wild plants. |
| |
4.4.11Define clone. |
|
Clone: a group of genetically identical organisms or a group of genetically identical cells derived from a single parent cell. |
| |
4.4.12 Outline a technique for cloning using differentiated animal cells. |
|
Dolly the sheep was cloned by taking udder cells from a donor sheep. These cells were than cultured in a low nutrient medium to make the genes switch off and become dormant. Then an unfertilized egg was taken from another sheep. The nucleus of this egg cell was removed by using a micropipette and then the egg cells were fused with the udder cells using a pulse of electricity. The fused cells developed like normal zygotes and became embryos. These embryos were then implanted into another sheep who’s role was to be the surrogate mother. One lamb was born successfully and called Dolly. Dolly was genetically identical to the sheep from which the udder cells were taken. |
| |
4.4.13 Discuss the ethical issues of therapeutic cloning in humans. |
|
Arguments for Arguments against Embryonic stem cells can be used for therapies that save lives and reduce pain for patients. Since a stem cell can divide and differentiate into any cell type, they can be used to replace tissues or organs required by patients. Every human embryo is a potential human being and should be given the chance of developing. Cells can be taken from embryos that have stopped developing and so these cells would have died anyway. More embryos are generally produced than are needed and so many are killed. Cells are taken at a stage when the embryos have no nerve cells and so they cannot feel pain. There is a risk of embryonic stem cells developing into tumour cells. |
| |
5.1.1 Define species, habitats, populations, community, ecosystems and ecology. |
|
Species: a group of organisms that can interbreed and produce fertile offspring. Habitat: the environment in which a species normally lives or the location of a living organism. Population: a group of organisms of the same species who live in the same area at the same time. Community: a group of populations living and interacting with each other in an area. Ecosystem: a community and its abiotic environment. Ecology: the study of relationships between living organisms and between organisms and their environment. |
| |
5.1.2 Distinguish between autotroph and heterotroph. |
|
Autotrophs are organisms that synthesize their organic molecules from simple inorganic substances whereas heterotrophs are organisms that obtain organic molecules from other organisms. |
| |
5.1.3 Distinguish between consumers, detritivores and saprotrophs. |
|
Consumer: an organism that ingests other organic matter that is living or recently killed. Detritivore: an organism that ingests non-living organic matter. Saprotroph: an organism that lives on or in non-living organic matter, secreting digestive enzymes into it and absorbing the products of digestion. |
| |
5.1.4 Describe what is meant by a food chain, giving three examples, each with at least three linkages (four organisms). |
|

A food chain shows the direction of energy flow from one species to another. For example, an arrow from A to B means that A is being eaten by B and therefore indicates the direction of the energy flow. |
| |
5.1.5 Describe what is meant by a food web. |
|
A food web is a diagram that shows all the feeding relationships in a community with arrows which show the direction of the energy flow. |
| |
5.1.6 Define trophic level. |
|
Trophic level: the trophic level of an organism is its position in the food chain. Producers, primary consumers, secondary consumers and tertiary consumers are examples of trophic levels. |
| |
5.1.7 Deduce the trophic level of organisms in a food chain and a food web. |
|
Plants or any other photosynthetic organisms are the producers. Primary consumers are the species that eat the producers. Secondary consumers are the species that eat the primary consumers and tertiary consumers in turn eat the secondary consumers. |
| |
5.1.8 Construct a food web containing up to 10 organisms, using appropriate information. |
|

 |
| |
5.1.9 State that light is the initial energy source for almost all communities. |
|
Light is the initial energy source for almost all communities. |
| |
5.1.10 Explain the energy flow in a food chain. |
|
Producers receive their energy from light energy (the sun) by means of photosynthesis. After this, the energy in organic matter flows from producers to primary consumers to secondary consumers to tertiary consumers. This is because producers will be eaten by primary consumers which in turn will be eaten by secondary consumers and so on. However, between these trophic levels, energy is always lost. All of the trophic levels lose energy as heat through cell respiration. Also, as the organic matter passes from one trophic level to the next, not all of it is digested and so we have loss of energy in organic matter through feces. This energy then passes on to the detritivores and saprotrophs. Another energy loss occurs through tissue loss and death which can happen at any trophic level. Once again, this energy would be passed on to detritivores and saprotrophs as they digest these. Detritivores and saprotrophs in turn lose energy as heat through cell respiration. |
| |
5.1.11 State that energy transformations are never 100% efficient. |
|
Energy transformations are never 100% efficient. |
| |
5.1.12 Explain that energy enters and leaves ecosystems, but nutrients must be recycled. |
|
Energy is not recycled. It is constantly being supplied to ecosystems through light energy and then flows through the trophic levels. As it flows through the trophic levels energy is lost in feces, tissue loss and death. This energy from these losses is passed on to detritivores and saprotrophs. However the energy is then lost from the ecosystem as the remaining energy in the trophic levels and the energy in the saprotrophs and detritivores is lost through cell respiration in the form of heat. As a result, energy needs to be constantly supplied to the ecosystems. Nutrients on the other hand are different as they constantly have to be recycled. Carbon, nitrogen and phosphorus are all examples of nutrients. There is only a limited supply of these as they are not resupplied to the ecosystems like energy. Therefor they have to be recycled over and over. They are absorbed from the environment, used by living organisms and then returned to the environment. |
| |
5.1.12 State that saprotrophic bacteria and fungi (decomposers) recycle nutrients. |
|
Saprotrophic bacteria and fungi (decomposers) recycle nutrients. |
| |
5.2.1 Draw and label a diagram of the carbon cycle to show the processes involved. |
|


The carbon cycle |
| |
5.2.2 Analyse the changes in concentration of atmospheric carbon dioxide using historical records. |
|
as time goes on increase, spike when fossil fuels begin being used |
| |
5.2.3 Explain the relationship between rises in concentrations of atmospheric carbon dioxide, methane and oxides of nitrogen and the enhanced greenhouse effect. |
|
The earths mean average temperature is regulated by a steady equilibrium which exists between the energy reaching the earth from the sun and the energy reflected by the earth back into space. The incoming radiation is short wave ultraviolet and visible radiation. Some of the radiation will be absorbed by the atmosphere and some of it will be reflected back from the earths surface into space. The radiation that is reflected back into space is infrared radiation which has a longer wavelength. Green house gases such as carbon dioxide, methane, and oxides of nitrogen tend to absorb some of the reflected infrared radiation and re-reflect it back towards the earth. This is what causes the greenhouse effect and it results in an increase in average mean temperature on earth. It is a natural phenomenon. However, since there has been an increase in the green house gases in the past century, this has resulted in an increase of the green house effect leading to higher than normal average temperatures which could lead to disastrous consequences in the future. |
| |
5.2.4 Outline the precautionary principle. |
|
The precautionary principle holds that, if the effects of a human-induced change would be very large, perhaps catastrophic, those responsible for the change must prove that it will not do harm before proceeding. This is the reverse of the normal situation, where those who are concerned about the change would have to prove that it will do harm in order to prevent such changes going ahead. |
| |
5.2.5 Evaluate the precautionary principle as a justification for strong action in response to the threats posed by the enhanced greenhouse effect. |
|
There is strong evidence that shows that green house gases are causing global warming. This is very worrying as global warming has so many consequences on ecosystems. If nothing is done, and the green house gases are in fact causing the enhanced green house effect, by the time we realize it, it will probably be too late and result in catastrophic consequences. So even though there is no proof for global warming, the strong evidence suggesting that it is linked with an increase in green house gases is something we can not ignore. Global warming is a global problem. It affects everyone. For these reasons, the precautionary principle should be followed. Anyone supporting the notion that we can continue to emit same amounts or more of the green house gases should have to provide evidence that it will not cause a damaging increase in the green house effect. |
| |
5.2.6 Outline the consequences of a global temperature rise on arctic ecosystems. |
|
Global warming could have a number of disastrous consequences largely affecting the arctic ecosystems: - The arctic ice cap may disappear as glaciers start to melt and break up into icebergs.
- Permafrost will melt during the summer season which will increase the rate of decomposition of trapped organic matter, including peat and detritus. This in turn will increase the release of carbon dioxide which will increase the green house effect even further.
- Species adapted to temperature conditions will migrate north which will alter food chains and have consequences on the animals in the higher trophic levels.
- Marine species in the arctic water may become extinct as these are very sensitive to temperature changes within the sea water.
- Polar bears may face extinction as they loose their ice habitat and therefore can no longer feed or breed as they normally would.
- Pests and diseases may become quite common with rises in temperature.
- As the ice melts, sea levels will rise and flood low lying areas of land.
- Extreme weather events such as storms might become common and have disastrous effects on certain species.
|
| |
5.3.1 Outline how population size is affected by natality, immigration, mortality and emigration. |
|
Natality: increases population size as offspring are added to the population. Immigration: increases population size as individuals have moved into the area from somewhere else and so this adds to the population. Mortality: decreases the population as some individuals get eaten, die of old age or get sick. Emigration: decreases the population as individuals have moved out of the area to go live somewhere else. |
| |
5.3.2 Draw and label a graph showing a sigmoid (S-shaped) population growth curve. |
|

|
| |
5.3.3 Explain the reasons for the exponential growth phase, the plateau phase and the transitional phase between these two phases. |
|
The sigmoid graph showing the population growth of a species has three phases which are; the exponential phase, the transitional phase and the plateau phase. At the start of the sigmoid curve we can see the exponential phase. This is where there is a rapid increase in population growth as natality rate exceeds mortality rate. The reason for this is because there are abundant resources available such as food for all members of the population and diseases as well as predators are rare. As time passes, the population reaches the transitional phase. This is where the natality rate starts to fall and/or the mortality rate starts to rise. It is the result of a decrease in the abundance of resources, and an increase in the number of predators and diseases. However, even though population growth has decreased compared to the exponential phase, it is still increasing as natality rate still exceeds mortality rate. Finally, the population reaches the plateau phase. Here, the population size is constant so no more growth is occurring. This is the result of natality rate being equal to mortality rate and is caused by resources becoming scarce as well as an increase in predators, diseases and parasites. These are the limiting factors to the population growth. If natality rate starts to drop then mortality rate will drop too as more resources become available. As natality rate starts to increase again so does mortality rate as resources become scarce. This keeps the population number relatively stable. If a population is limited by a shortage of resources then we say that it has reached the carrying capacity of the environment. |
| |
5.3.4 List three factors that set limits to population increase. |
|
- Shortage of resources (e.g. food)
- Increase in predators
- Increase in diseases and parasites
|
| |
5.4.1 Define evolution |
|
Evolution is the cumulative change in the heritable characteristics of a population. |
| |
5.4.2 Outline the evidence for evolution provided by the fossil record, selective breeding of domesticated animals and homologous structures. |
|
Fossils, selective breeding and homologous structures have provided scientists with evidence that support the theory of evolution. As they started to study fossils they realised that these were not identical but had similarities with existing organisms. This suggested that organisms changed over time. Selective breeding of domesticated animals also provides this evidence as the domestic breeds have similar characteristics to the wild ones and can still breed with them. As selected wild individuals with desirable characteristics were bred, over time this resulted in a more desirable species from a human point of view. An example of this is the taming of wild wolves and their selective breeding in order to produce the domestic dogs we know today. This suggests that not only have these animals evolved but also that they can evolve rapidly. Finally scientists have found a number of homologous structures within different species. Many bones in the limbs are common to a number of species and therefore suggests that these have evolved from one common ancestor. |
| |
5.4.3 State that populations tend to produce more offspring than the environment can support. |
|
Populations tend to produce more offspring than the environment can support. |
| |
5.4.4 Explain that the consequence of the potential over production of offspring is a struggle for survival. |
|
If the mortality rate remains lower than the natality rate then a population will keep growing. As more offspring are produced, there will be less resources available to other members of the population. If there is an over production of offspring this will result in a struggle for survival within the species as the resources become scarce and individuals in the population will start to compete for these. This results in an increase in mortality rate as the weaker individuals in the population will lose out on these vital resources that are essential for their survival. |
| |
5.4.5 State that members of a species show variation. |
|
Members of a species show variation. |
| |
5.4.6 Explain how sexual reproduction promotes variation in a species. |
|
Sexual reproduction is important for promoting variation as even though mutations form new genes or alleles, sexual reproduction forms a new combination of alleles. There are two stages in sexual reproduction that promote variation in a species. The first one is during meiosis during which a large variety of genetically different gametes are produced by each individual. The second stage is fertilisation. Here, alleles from two different individuals are brought together to form one new individual. |
| |
5.4.7 Explain how natural selection leads to evolution. |
|
Individuals in a population differ from each other. Some individuals will have characteristics that make them well adapted to their environment whereas others will have characteristics that make them less adapted to their environment. The better adapted individuals are the ones that are more likely to survive and produce offspring while the less adapted ones are more likely to die. This is called natural selection. Natural selection results in the better adapted individuals to pass on their characteristics to more offspring as the lesser adapted ones are more likely to die before they reproduce. Over time, this result accumulates and a new generation is created with the favourable characteristics that makes this species better adapted to its environment. Natural selection has lead to the species evolving. |
| |
5.4.8 Explain two examples of evolution in response to environmental change; one must be antibiotic resistance in bacteria. |
|
Antibiotic resistance in bacteria is a common problem. It results from the transfer of a gene that gives resistance to a specific antibiotic usually by means of a plasmid to a bacterium. Some bacteria will then have this gene and become resistant to the specific antibiotic while others will lack the gene and so will die if exposed to the antibiotic. Over time, the non-resistant ones will all die off as doctors vaccinate patients, but the resistant ones will survive. Eventually, the resistant ones will be the only ones left as a result of natural selection and so a new antibiotic must be created. However, this has to be done on a regular basis as the bacteria keep evolving and become resistant to multiple antibiotics. The Peppered Moth is another example of evolution in response to environmental change. There are two types of these moths, one species has a light colour while the other one is darker. When Britain begun industrialising, the soot from the factories would land on trees and so the darker moths then had an advantage over the light ones as they could easily hide from predators. Before the soot, both types of moths were eaten by predators however now that the darker ones were able to hide the lighter ones got eaten more often.The population of the darker moths rapidly increased while that of the lighter ones rapidly decreased until only the dark moths were left. All the lighter moths were less adapted to the environmental change and so they could no longer survive in that new environment. |
| |
6.1.1 Explain why digestion of large food molecules is essential. |
|
There are two reasons why the digestion of large food molecules is vital. Firstly, the food we eat is made up of many compounds made by other organisms which are not all suitable for human tissues and therefore these have to be broken down and reassembled so that our bodies can use them. Secondly, the food molecules have to be small enough to be absorbed by the villi in the intestine through diffusion, facilitated diffusion or active transport and so large food molecules need to be broken down into smaller ones for absorption to occur. |
| |
6.1.2 Explain the need for enzymes in digestion. |
|
Enzymes are needed in the process of digestion as they are the biological catalysts which break down the large food molecules into smaller ones so that these can eventually be absorbed. Digestion can occur naturally at body temperature, however this process takes a very long time as it happens at such a slow rate. For digestion to increase in these circumstances, body temperature would have to increase as well. However this is not possible as it would interfere with other body functions.This is why enzymes are vital as they speed up this process by lowering the activation energy required for the reaction to occur and they do so at body temperature. |
| |
6.1.3 State the source, substrate, products and optimum pH conditions for one amylase, one protease and one lipase. |
|
Amylase Protease Lipase Enzyme Salivary Amylase Pepsin Pancreatic Lipase Source Salivary Glands Chief cells in stomach lining Pancreas Substrate Starch Proteins Triglycerides such as fats and oils Products Maltose Small polypeptides Fatty Acids and Glycerol Optimum pH pH 7 pH 1.5 - 2 pH 7 |
| |
6.1.4 Draw and label a diagram of the digestive system. |
|

 |
| |
6.1.5 Outline the functions of the stomach, small intestine and large intestine. |
|
The stomach is an important part of the digestive system. Firstly it secretes HCL which kills bacteria and other harmful organisms preventing food poisoning and it also provides the optimum conditions for the enzyme pepsin to work in (pH 1.5 - 2). In addition, the stomach secretes pepsin which starts the digestion of proteins into polypeptides and amino acids. Theses can then be absorbed by the villi in the small intestine. The small intestine is where the final stages of digestion occur. The intestinal wall secretes enzymes and it also receives enzymes from the pancreas. However the main function of the small intestine is the absorption of the small food particles resulting from digestion. It contains many villi which increase the surface area for absorption. The large intestine moves the material that has not been digested from the small intestine and absorbs water. This produces solid faeces which are then egested through the anus. |
| |
6.1.6 Distinguish between absorption and assimilation. |
|
Absorption occurs when the food enters the body as the food molecules pass through a layer of cells and into the bodies tissues. This occurs in the small intestine which has many villi that are specialised for absorption. Assimilation occurs when the food molecules becomes part of the bodies tissue. Therefore, absorption is followed by assimilation. |
| |
6.1.7 Explain how the structure of the villus is related to its role in absorption and transport of the products of digestion. |
|

The structure of the villus is very specific. Firstly there is a great number of them so this increases the surface area for absorption in the small intestine. In addition the villi also have their own projections which are called microvilli. The many microvilli increase the surface area for absorption further. These microvilli have protein channels and pumps in their membranes to allow the rapid absorption of food by facilitated diffusion and active transport. Also, the villi contains an epithelial layer which is only one cell layer thick so that food can pass through easily and be absorbed quickly. The blood capillaries in the villus are very closely associated with the epithelium so that the distance for the diffusion of the food molecules is small. This thin layer of cells contains mitochondria to provide the ATP needed for the active transport of certain food molecules. Finally, there is a lacteal branch at the centre of the villus which carries away fats after absorption.

|
| |
6.2.1 Draw and label a diagram of the heart showing the four chambers, associated blood vessels, valves and the root of the blood through the heart. |
|

 |
| |
6.2.2 State that the coronary arteries supply heart muscle with oxygen and nutrients. |
|
The coronary arteries supply heart muscle with oxygen and nutrients. |
| |
6.2.3 Explain the actions of the heart in terms of collecting blood, pumping blood and opening and closing valves. |
|
The right atrium collects blood from the superior and inferior vena cava and the left atrium collects blood from the pulmonary veins. This blood then flows into the right and left ventricle which pump the blood into the arteries. The direction of the blood flow is controlled by the atrioventricular valves and semilunar valves. When the atria contract the blood flows through the atrioventricular valves which are open, into the ventricle. At this stage the semilunar valves are closed so the ventricle fills with blood. The ventricles then contract which causes a rise in pressure. This rise in pressure first causes the atrioventricular valves to close preventing back flow of blood into the atria. Then the semilunar valves open allowing the expulsion of blood into the arteries. As this happens, the atria start to fill with blood again. The ventricles stop contracting leading to a fall in pressure which causes the semilunar valves to close, preventing back flow of blood from the arteries. When the ventricular pressure drops below the atrial pressure the atrioventricular valves open again and the cycle repeats. |
| |
6.2.4 Outline the control of the heartbeat in terms of myogenic muscle contraction, the role of the pacemaker, nerves, the medulla of the brain and epinephrine (adrenaline). |
|
The heart muscle can contract by itself, without the stimulation of a nerve. This is called myogenic muscle contraction. The region that initiates each contraction is found in the wall of the right atrium and is called the pacemaker. Every time the pacemaker sends out a signal, a heartbeat results. The pacemaker is under the influence of nerves and adrenaline. One nerve carries messages from the medulla of the brain to the pacemaker and speeds up the beating of the heart. Another nerve carries messages from the medulla of the brain to the pacemaker and slows down the beating of the heart. Finally, adrenaline (epinephrine) is carried by the blood and once it reaches the pacemaker it signals it to increase the beating of the heart. |
| |
6.2.5 Explain the relationship between the structure and function of arteries, capillaries and veins. |
|
Arteries have a thick outer layer of longitudinal collagen and elastic fibers to avoid leaks and bulges. They have a thick wall which is essential to withstand the high pressures. They also have thick layers of circular elastic fibres and muscle fibres to help pump the blood through after each contraction of the heart. In addition the narrow lumen maintains the high pressure inside the arteries. Veins are made up of thin layers with a few circular elastic fibres and muscle fibres. This is because blood does not flow in pulses and so the vein walls cannot help pump the blood on. Veins also have thin walls which allows the near by muscles to press against them so that they become flat. This helps the blood to be pushed forwards towards the heart. There is only a thin outer layer of longitudinal collagen and elastic fibres as there is low pressure inside the vein and so little chance of bursting. Finally, a wide lumen is needed to accommodate the slow flowing blood due to the low pressure. Capillaries are made up of a wall that is only one cell layer thick and results in the distance for diffusion in and out of the capillary being very small so that diffusion can occur rapidly. They also contain pores within the their wall which allow some plasma to leak out and form tissue fluid. Phagocytes can also pass through these pores to help fight infections. In addition, the lumen of the capillaries is very narrow. This means that many capillaries can fit in a small space, increasing the surface area for diffusion. |
| |
6.2.6 State that blood is composed of plasma, erythrocytes, leucocytes (phagocytes and lymphocytes) and platelets. |
|
Blood is composed of plasma, erythrocytes, leucocytes (phagocytes and lymphocytes) and platelets. |
| |
6.2.7 State that the following are transported by the blood: nutrients, oxygen, carbon dioxide, hormones, antibodies, urea and heat. |
|
Nutrients, oxygen, carbon dioxide, hormones, antibodies, urea and heat are all transported by the blood. |
| |
6.3.1 Define pathogen. |
|
Pathogen: an organism or virus that causes a disease. |
| |
6.3.2 Explain why antibiotics are effective against bacteria but not against viruses. |
|
Antibiotics are produced by microorganisms to kill or control the growth of other microorganisms by blocking specific metabolic pathways within the cell. Since bacteria are so different to human cells, antibiotics can be taken by humans to kill bacteria without harming the human cells. Viruses on the other hand are different as they do not carry out many metabolic processes themselves. Instead they rely on a host cell (a human cell) to carry out these processes for them. Therefore viruses cannot be treated with antibiotics as it is impossible to harm the virus without harming the human cells. |
| |
6.3.3 Outline the role of skin and mucous membranes in the defence against pathogens. |
|
The skin forms a physical barrier that prevents pathogens from entering the body as the outer layer is very tough. In addition the skin contains sebaceous glands which secret lactic acid and fatty acids which creates an acidic environment on the surface of the skin preventing the growth of pathogens. Mucous membranes form another type of barrier against pathogens. Mucous membranes are soft and moist areas of skin found in the trachea, nose, vagina and urethra. These membranes are not strong enough to create a physical barrier but they do have mucus which contain lysozyme enzymes that digest the phagocytes. Also, the mucus can be sticky such as in the trachea, and trap the pathogens which are then expelled up the trachea and out of the body by muscles within the trachea. |
| |
6.3.4 Outline how phagocytic leucocytes ingest pathogens in the blood and body tissues. |
|
Phagocytes are found in the blood and ingest pathogens. They do so by recognising pathogens and engulfing them by endocytosis. Enzymes within the phagocytes called lysosomes then digest the pathogens. Phagocytes can ingest pathogens in the blood but also within body tissue as they can pass through the pores of capillaries and into these tissues. |
| |
6.3.5 Distinguish between antigens and antibodies. |
|
Antibodies are proteins that defend the body against pathogens by binding to antigens on the surface of these pathogens and stimulating their destruction. Antigens are foreign substances which stimulate the production of antibodies. Antibodies usually only bind to one specific antigen. |
| |
6.3.6 Explain antibody production. |
|
Lymphocytes are a type of leukocyte which make antibodies. Each lymphocyte makes only one specific antibody. A large amount of different lymphocytes are needed so that the body can produce different types of antibodies. The antibodies are found on the surface of the plasma membrane of these lymphocytes with the antigen-combining site projecting outwards. Pathogens have antigens on their surface which bind to the antigen-combining site of the antibodies of a specific lymphocyte. When this happens the lymphocyte becomes active and starts to make clones of itself by dividing by mitosis. These clones then start to make more of this specific antibody needed to defend the body against the pathogen. |
| |
6.3.7 Outline the effects of HIV on the immune system. |
|
The HIV virus (which causes AIDS) destroys a type of lymphocyte which has a vital role in antibody production. Over the years this results in a reduced amount of active lymphocytes. Therefore, less antibodies are produced which makes the body very vulnerable to pathogens. A pathogen that could easily be controlled by the body in a healthy individual can cause serious consequences and eventually lead to death for patients affected by HIV. |
| |
6.3.8 Discuss the cause, transmission and social implications of AIDS. |
|
Cause: HIV causes AIDS (acquired immunodeficiency syndrome). A syndrome is a group of symptoms that are found together. HIV destroys a type of lymphocyte which is vital for antibody production. Over the years, less active lymphocytes are produced which leads to a fall in the amount of antibodies. Pathogens that would normally be easily controlled by the body in healthy individuals can cause serious consequences and eventually lead to death for patients affected by HIV. The immune system is considerably weakened. Transmission: HIV is transmitted through body fluids from an infected person to an uninfected one. This can occur through vaginal and anal intercourse as well as oral sex if there are cuts or tears in the vagina, penis, mouth or intestine. It can also be transmitted by hypodermic needles that are shared by intravenous drug abusers. The small amount of blood present on these needles after their use may contain the virus and is enough to infect another person. Another way of transmission is through the placenta from mother to child, or through cuts during childbirth or in milk during breast feeding. Finally there is a risk of transmission in transfused blood or with blood products such as Factor VIII used to treat hemophiliacs. Social implications: Relatives and friends suffer grief. Families can also suffer from a loss of income as the person infected by HIV can lose their wage if they are unable to work and are refused life insurance. Also, HIV patients may find it hard to find partners, employment and even housing. Finally, AIDS can cause fear in a population and reduce sexual activity. |
| |
6.4.1 Distinguish between ventilation, gas exchange and cell respiration. |
|
Ventilation is the process of bringing fresh air into the alveoli and removing the stale air. It maintains the concentration gradient of carbon dioxide and oxygen between the alveoli and the blood in the capillaries (vital for oxygen to diffuse into the blood from the alveoli and carbon dioxide out of the blood into the alveoli). Gas exchange is the process of swapping one gas for another. It occurs in the alveoli of the lungs. Oxygen diffuses into the capillaries from the air in the alveoli and carbon dioxide diffuses out of the capillaries and into the air in the alveoli. Cell respiration releases energy in the form of ATP so that this energy can be used inside the cell. Cell respiration occurs in the mitochondria and cytoplasm of cells. Oxygen is used in this process and carbon dioxide is produced. |
| |
6.4.2 Explain the need for a ventilation system |
|
A ventilation system is needed to maintain the concentration gradients of gases in the alveoli. Diffusion of gases occurs due to the concentration gradient of oxygen and carbon dioxide between the alveoli and the blood. The body needs to get rid of carbon dioxide which is a product of cell respiration and needs to take in oxygen as it is needed for cell respiration to make ATP. There must be a low concentration of carbon dioxide in the alveoli so that carbon dioxide can diffuse out of the blood in the capillaries and into the alveoli. Also there must be a high concentration of oxygen in the in the alveoli so that oxygen can diffuse into the blood in the capillaries from the alveoli. The ventilation system makes this possible by getting rid of the carbon dioxide in the alveoli and bringing in more oxygen. |
| |
6.4.3 Describe the features of alveoli that adapt them to gas exchange. |
|
Even though alveoli are so small there are huge numbers of them which results in a large surface area for gas exchange. Also the wall of the alveoli is made up of a single layer of thin cells and so are the capillaries, this creates a short diffusion distance for the gases. Therefore this allows rapid gas exchange. The alveoli are covered by a dense network of blood capillaries which have a low oxygen and high carbon dioxide concentrations. This allows oxygen to diffuse into the blood and carbon dioxide to diffuse out of the blood. Finally, there are cells in the alveolar walls which secrete a fluid that keeps the inner surface of the alveoli moist, allowing gases to dissolve. This fluid also contains a natural detergent that prevents the sides of the alveoli from sticking together. |
| |
6.4.4 Draw and label a diagram of the ventilation system, including trachea, lungs, bronchi, bronchioles and alveoli. |
|
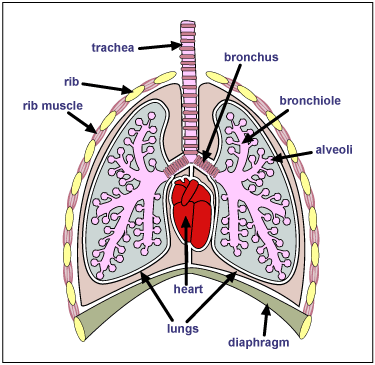
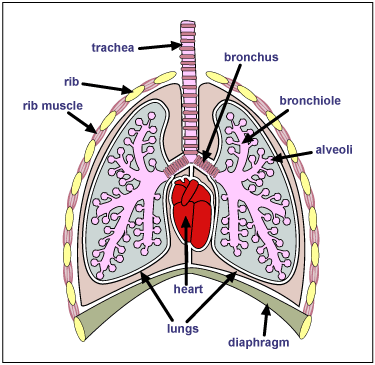 |
| |
6.4.5 Explain the mechanism of ventilation of the lungs in terms of volume and pressure changes caused by the internal and external intercostal muscles, the diaphragm and the abdominal muscles. |
|
Inhalation: - The external intercostal muscles contract. This moves the ribcage up and out. - The diaphragm contracts. As it does so it moves down and becomes relatively flat. - Both of these muscle contractions result in an increase in the volume of the thorax which in turn results in a drop in pressure inside the thorax. - Pressure eventually drops below atmospheric pressure. - Air then flow into the lungs from outside the body, through the mouth or nose, trachea, bronchi and bronchioles. - Air continues to enter the lungs until the pressure inside the lungs rises to the atmospheric pressure. Exhalation: - The internal intercostal muscles contract. This moves the ribcage down and in. - The abdominal muscles contract. This pushes the diaphragm up, back into a dome shape. - Both of these muscle contractions result in a decrease in the volume of the thorax. - As a result of the decrease in volume, the pressure inside the thorax increases. - Eventually the pressure rises above atmospheric pressure. - Air then flows out of the lungs to outside of the body through the nose or mouth. - Air continues to flow out of the lungs until the pressure in the lungs has fallen back to atmospheric pressure. |
| |
6.5.1 State that the nervous system consists of the central nervous system (CNS) and peripheral nerves, and is composed of cells called neurons that can carry rapid electrical impulses. |
|
The nervous system consists of the central nervous system (CNS) and peripheral nerves, and is composed of cells called neurons which carry rapid electrical impulses. |
| |
6.5.2 Draw and label a diagram of the structure of a motor neuron. |
|

 |
| |
6.5.3 State that nerve impulses are conducted from receptors to the CNS by sensory neurons, within the CNS by relay neurons, and from the CNS to effectors by motor neurons. |
|
Nerve impulses are conducted from receptors to the CNS by sensory neurons, within the CNS by relay neurons, and from the CNS to effectors by motor neurons. |
| |
6.5.4 Define resting potential and action potential (depolarization and repolarization). |
|
Resting potential: the electrical potential across the plasma membrane of a cell that is not conducting an impulse. Action potential: the reversal and restoration of the electrical potential across the plasma membrane of a cell, as an electrical impulse passes along it (depolarization and repolarization). |
| |
6.5.5 Explain how a nerve impulse passes along a non-myelinated neuron. |
|
Sodium is found in greater concentrations outside of the cell while potassium is found in greater concentrations inside the cell. Sodium-potassium pumps exist in the plasma membrane to maintain the the concentration gradients and the membrane potential. Nerve impulses have a domino effect. An action potential in one part of the neuron causes another action potential in the adjacent part and so on. This is due to the diffusion of sodium ions between the region of the action potential and the resting potential. It is the movement of sodium and potassium that reduce the resting potential. If the resting potential rises above the threshold level, voltage gated channels open. Voltage gated sodium channels open very fast so that sodium can diffuse into the cell down its concentration gradient. This reduces the membrane potential and results in more sodium channels opening. Sodium ions are positively charged and so the inside of the cell develops a net positive charge compared to the outside of the cell. This results in depolarization as the potential across the membrane is reversed. A short while after this, voltage gated potassium channels open and potassium ions flow out of the cell down the concentration gradient. Since potassium ions are positively charged, their diffusion out of the cell causes a net negative charge to develop again inside the cell compared to the outside. The potential across the membrane is restored. This is called repolarization. Finally, the concentration gradients of both ions are restored by the sodium-potassium pump. Sodium is pumped out of the cell while potassium is pumped in. The resting potential is restored and the neuron is ready to conduct another nerve impulse. |
| |
6.5.6 Explain the principles of synaptic transmissions. |
|

A synapse is a junction that permits a neuron to pass an electrical or chemical signal to another cell. At a synapse, the plasma membrane of the signal passing neuron (presynaptic neuron) is closely related to the plasma membrane of the target cell (postsynaptic neuron). Between the two there is a narrow fluid filled space called the synaptic cleft. Chemical signals called neurotransmitters pass from the presynaptic neuron to the post synaptic neuron. This is how a synaptic transmission occurs: An action potential travels along the neuron and reaches the end of the pre-synaptic neuron. The depolarization of the pre-synaptic membrane results in the opening of voltage gated calcium channels. Calcium ions flow into the presynaptic neuron and cause vesicles with neurotransmitters inside the neuron to fuse with the plasma membrane and release the neurotransmitters into the synaptic cleft via exocytosis. These neurotransmitters then diffuse within the synaptic cleft and some will bind to specific receptors located on the postsynaptic plasma membrane. The receptors are transmitted-gated ion channels which open and let sodium and other positively charged ions into the postsynaptic neuron when the neurotransmitters bind. As these positively charged ions enter the postsynaptic neuron they cause its membrane to depolarize. This depolarization results in an action potential which passes down the postsynaptic neuron. The neurotransmitters in the synaptic cleft are then quickly degraded and the calcium ions are pumped back into the synaptic cleft from inside the presynaptic neuron.

|
| |
6.5.7 State that endocrine systems consist of glands that release hormones that are transported in the blood. |
|
The endocrine system consists of glands that release hormones that are transported in the blood. |
| |
6.5.8 State that homeostasis involves maintaining the internal environment between limits, including blood pH, carbon dioxide concentration, blood glucose concentration, body temperature and water balance. |
|
Homeostasis involves maintaining the internal environment between limits, including blood pH, carbon dioxide concentration, blood glucose concentration, body temperature and water balance. |
| |
6.5.9 Explain that homeostasis involves monitoring levels of variables and correcting changes in levels by negative feedback mechanisms. |
|
Homeostasis involves maintaining the internal environment between limits, including blood pH, carbon dioxide concentration, blood glucose concentration, body temperature and water balance. Blood and tissue fluid (derived from blood) make up the internal environment. This internal environment varies very little compared to the external environment which varies greatly. Negative feed back is used to keep the internal environment between limits. It uses the nervous and endocrine system to do so. It has a stabilising effect as any change from a set point level will result in an opposite change. The levels of production of for example blood glucose, feed back to affect the rate of production. If blood glucose levels rise above the set point, this will feed back to decrease production and reduce the level back around the set point. A decrease in blood glucose levels below the set point will result in an increase in production so that the levels increase back to the set point. Small fluctuations around the set point will not cause any response. Negative feed back is only triggered when there are significant increases or decreases from the set point. |
| |
6.5.10 Explain the control of body temperature, including the transfer of heat in the blood, and the roles of the hypothalamus, sweat glands and skin, arterioles and shivering. |
|
he hypothalamus is responsible for monitoring the temperature of the blood which is normally close to 37 degrees. If there are significant fluctuations from this set point, the hypothalamus sends signals (messages carried by neurons) to different parts of the body to restore the temperature back to the set point. This is done through negative feedback. If blood temperature significantly increases above the set point If blood temperature significantly drops bellow the set point Skin arterioles increase in diameter so that more blood flows to the skin. By doing so it transfers heat from the core of the body to the skin and this heat is then lost to the external environment, cooling down the body in the process. Skin arterioles decrease in diameter so that less blood flows to the skin. The diameter of the capillaries in the skin cannot change but less blood flows through them. This prevents heat loss to the external environment as the temperature of the skin falls. Skeletal muscle stays relaxed so that more heat is not generated. Shivering occurs. This is when the skeletal muscle does many small rapid contractions to generate heat. Sweat glands secrete large amounts of sweat which makes the surface of the skin moist. When water evaporates from the moist skin it cools down the body. Sweat glands to not secrete sweat and so no water evaporation can occur as skin stays dry. |
| |
6.5.11 Explain the control of blood glucose concentration, including the role of glucagon, insulin and α and ß cells in the pancreatic islets. |
|
Blood glucose concentration does not have a specific set point like blood temperature. Blood glucose levels drop and rise through the day and so the body usually tries to keep blood glucose levels around 4 to 8 millimoles per dm3 of blood. Once again, negative feedback is used to do so. There are responses by target organs which affect the rate at which glucose is taken up from the blood or loaded into the blood. Response to blood glucose levels above the set point Response to blood glucose levels below the set point β cells in the pancreatic islets produce insulin. Insulin stimulates muscle cells and the liver cells to take up glucose from the blood and convert it into glycogen. These are then stored in the form of granules in the cytoplasm of cells. Also, other types of cells are stimulated to take up glucose and use it for cell respiration instead of fat. All of these processes lower the levels of glucose in the blood. α cells in the pancreatic islets produce glucagon. Glucagon stimulates the liver cells to convert glycogen back into glucose and release this glucose into the blood. This raises the glucose levels in the blood. |
| |
6.5.12 Distinguish between type I and type II diabetes. |
|
Type I diabetes Type II diabetes The onset is usually early, sometime during childhood. The onset is usually late, sometime after childhood. β cells do not produce enough insulin. Target cells become insensitive to insulin. Diet by itself cannot be used to control the condition. Insulin injections are needed to control glucose levels. Insulin injections are not usually needed. Low carbohydrate diet can control the condition. |
| |
6.6.1 Draw and label diagrams of the adult male and female reproductive systems. |
|


 |
| |
6.6.2 Outline the role of hormones in the menstrual cycle, including FSH (follicle stimulating hormones), LH (luteinizing hormone), estrogen and progesterone. |
|
The menstrual cycle: - FSH is secreted by the pituitary gland and its levels start to rise. This stimulates the follicle to develop and the follicle cells to secret estrogen.
- Estrogen then causes the follicle cells to make more FSH receptors so that these can respond more strongly to the FSH.
- This is positive feedback and causes the estrogen levels to increase and stimulate the thickening of the endometrium (uterus lining).
- Estrogen levels increase to a peak and by doing so it stimulates LH secretion from the pituitary gland.
- LH then increases to its peak and causes ovulation (release of egg from the follicle).
- LH then stimulates the follicle cells to secrete less estrogen and more progesterone. Once ovulation has occurred, LH stimulated the follicle to develop into the corpus luteum.
- The corpus luteum then starts to secrete high amounts of progesterone. This prepares the uterine lining for an embryo.
- The high levels of estrogen and progesterone then start to inhibit FSH and LH.
- If no embryo develops the levels of estrogen and progesterone fall. This stimulates menstruation (break down of the uterine lining). When the levels of these two hormones are low enough FSH and LH start to be secreted again.
- FSH levels rise once again and a new menstrual cycle begins.
|
| |
6.6.3 Annotate a graph showing hormone levels in the menstrual cycle, illustrate the relationship between changes in hormone levels and ovulation, menstruation and the thickening of the endometrium. |
|

|
| |
6.6.4 List three roles of testosterone in males. |
|
Roles: - Stimulates the development of prenatal genitalia.
- Stimulates the development of the male secondary sexual characteristics such as growth of the skeletal muscle and pubic hair.
- During adulthood it maintains the sex drive.
|
| |
6.6.5 Outline the process of in vitro fertilization (IVF). |
|
Process: - For a period of three weeks, the women has to have a drug injected to stop her normal menstrual cycle.
- After these three weeks, high doses of FSH are injected once a day for 10-12 days so that many follicles develop in the ovaries of the women.
- HCG (another hormone) is injected 36 hours before the collection of the eggs. HCG loosens the eggs in the follicles and makes them mature.
- The man needs to ejaculate into a jar so that sperm can be collected from the semen. The sperm are processed to concentrate the healthiest ones.
- A device that is inserted through the wall of the vagina is used to extract the eggs from the follicles.
- Each egg is then mixed with sperm in a shallow dish. The dishes are then put into an incubator overnight.
- The next day the dishes are looked at to see if fertilization has happened.
- If fertilization has been successful, two or three of the embryos are chosen to be placed in the uterus by the use of a long plastic tube.
- A pregnancy test is done a few weeks later to find out if any of the embryos have implanted.
- A scan is done a few weeks later to find out if the pregnancy is progressing normally.
|
| |
6.6.6 Discuss the ethical issues associated with IVF. |
|
Arguments for IVF Arguments against IVF Many types of infertility are due to environmental factors rather than genetic which means that the offspring would not inherit the infertility. The infertility of the parents may be inherited by their offspring passing on the suffering to the next generation. The embryos that are killed during the IVF process cannot feel pain or suffering as they do not have a developed nervous system. More embryos are produced than needed and the ones that remain are usually killed which denies them the chance of a life. Suffering caused by genetic diseases can be decreases by screening the embryos before placing them into the uterus. Embryologists select which embryos will be placed into the uterus. Therefore they decide the fate of new individuals as they choose which ones will survive and which ones will die. Since the IVF process is not an easy one emotionally and physically, is costly, takes time and there are no guarantees, parents who are willing to go through it must have a strong desire to have children and therefore are likely to be loving parents. IVF is not a natural process which takes place in a laboratory compared to natural conception which occurs as a result of an act of love. Infertility can cause emotional suffering to couples who want to have children. IVF can take away this suffering for some of those couples. Infertility should be accepted as God’s will and to go against it by using IVF procedures would be wrong. |
| |
7.1.1 Describe the structure of DNA, including the antiparallel strands, 3'-5' linkages and hydrogen bonding between purines and pyrimidines. |
|
DNA is made up of two strands. At one end of each strand there is a phosphate group attached to the carbon atom number 5 of the deoxyribose (this indicates the 5' terminal) and at the other end of each strand is a hydroxyl group attached to the carbon atom number 3 of the deoxyribose (this indicates the 3' terminal). The strands run in opposite directions and so we say that they are antiparallel. One strand runs in a 5'-3' direction and the other runs in a 3'-5' direction. Adjacent nucleotides are attached together via a bond between the phosphate group of one nucleotide and the carbon atom number 3 of the deoxyribose of the other nucleotide. The bases of each strand link together via hydrogen bonds. Adenine and Guanine are purines as they have two rings in their molecular structure. Thymine and Cytosine are pyrimidines as they only have one ring in their molecular structure. A purine will link with a pyrimidine. Adenine and thymine link together by forming two hydrogen bonds while Guanine and cytosine link together by forming 3 hydrogen bonds. |
| |
7.1.2 Outline the structure of nucleosomes. |
|
Nucleosomes consiste of DNA wrapped around eight histone proteins and held together by another histone protein. |
| |
7.1.3 State that nucleosomes help to supercoil chromosomes and help to regulate transcription. |
|
Nucleosomes help to supercoil chromosomes and help regulate transcription. |
| |
7.1.4 Distinguish between unique or single-copy genes and highly repetitive sequences in nuclear DNA. |
|
Not all of the base sequences in DNA are translated. Highly repetitive base sequences are not translated. They consist of sequences of between 5 and 300 bases that may be repeated up to 10 000 times. They constitute 5-45% of eukaryotic DNA. Single-copy genes or unique genes are translated and constitute a surprisingly small proportion of eukaryotic DNA. |
| |
7.1.5 State that eukaryotic genes can contain exons and introns. |
|
Eukaryotic genes can contain exons and introns. |
| |
7.2.1 State that DNA replication occurs in a 5'→3' direction. |
|
DNA replication occurs in a 5'→3' direction. |
| |
7.2.2 Explain the process of DNA replication in prokaryotes, including the role of enzymes (helicase, DNA polymerase, RNA primase and DNA ligase), Okazaki fragments and deoxynucleoside triphosphate. |
|
The first stage of DNA replication in prokaryotes is the uncoiling of the DNA double helix by the enzyme helicase. Helicase separates the DNA into two template strands. RNA primase then adds a short sequence of RNA to the template strands. This short sequence of RNA is a primer which allows DNA polymerase III to bind to the strands and start the replication process. Once this is done, DNA polymerase III adds nucleotides to each template strand in a 5'→3' direction. The nucleotides have 3 phosphate groups and are called deoxyribonucleoside triphosphates. Two of these phosphate groups break off during the replication process to release energy. Since the strands are anti-parallel (the two strands have their 5' end and 3' end in opposite sides) and replication can only occur in a 5'→3' direction, one of the strands will be replicated in the same direction as the replication fork and the other will be replicated in the opposite direction of the replication fork. This means that one of the strands is synthesised in a continuous manner (named the leading strand) while the other one is synthesised in fragments (named the lagging strand). The leading strand only needs one primer while the lagging strand needs quite a few as it is formed in fragments. These fragments are called Okazaki fragments. DNA polymerase I will remove the RNA primers and replace these with DNA. The enzyme DNA ligase then joins the Okazaki fragments together to form a continuous strand. |
| |
7.2.3 State that DNA replication is initiated at many points in eukaryotic chromosomes. |
|
DNA replication is initiated at many points in eukaryotic chromosomes. |
| |
7.3.1 State that transcription is carried out in a 5'→3' direction. |
|
Transcription is carried out in a 5'→3' direction. |
| |
7.3.2 Distinguish between the sense and antisense strands of DNA. |
|
The antisense strand is the template DNA strand which is transcribed. The sense strand on the other hand is the DNA strand which has the same base sequence as the mRNA with thymine instead or uracil. |
| |
7.3.3 Explain the process of transcription in prokaryotes, including the role of the promoter region, RNA polymerase, nucleoside triphosphates and the terminator. |
|
mRNA is produced during transcription. In prokaryotes, RNA polymerase recognises a specific sequence of DNA called the promoter. The promoter basically "tells" the RNA polymerase where to start the transcription process. Transcription is initiated with the binding of RNA polymerase to the promoter site. The RNA polymerase then uncoils the DNA and separates the two strands. One of the strands is used as the template strand for transcription. The RNA polymerase will then use free nucleoside triphosphates to build the mRNA in a 5'→3' direction. These nucleoside triphosphates bond to their complementary base pairs on the template strand. As they bind they become nucleotides by losing two phosphate groups to release energy. Since RNA does not contain thymine, uracil pairs up with adenine instead. RNA polymerase forms covalent bonds between these nucleotides. It moves along the DNA to keep elongating the sequence of mRNA until it reaches a sequence of DNA called the terminator. This sequence of DNA "tells" the RNA polymerase to stop transcription. The RNA polymerase is then released from the DNA and the newly created mRNA separates from the template DNA strand. Finally, the DNA rewinds back to its original double helical structure. |
| |
7.3.4 State that eukaryotic RNA needs the removal of introns to form mature mRNA |
|
Eukaryotic RNA needs the removal of introns to form mature mRNA. |
| |
7.4.1 Explain that each tRNA molecule is recognised by a tRNA-activating enzyme that binds a specific amino acid to the tRNA, using ATP for energy. |
|
There are many different types of tRNA and each tRNA is recognised by a tRNA-activating enzyme. This enzyme binds a specific amino acid to the tRNA by using ATP as an energy source. The tRNA molecule has a specific structure. It contains double stranded sections (due to base pairing via hydrogen bonds) and loops. It has an anticodon loop which contains the anticodon and two other loops. The nucleotide sequence CCA is found at the 3' end of the tRNA and allows attachment for an amino acid. Each type of tRNA has slightly different chemical properties and three dimensional structure which allows the tRNA-activating enzyme to attach the correct amino acid to the 3' end of the tRNA. There are 20 different tRNA-activating enzymes as there are 20 different amino acids. Each enzyme will attach a specific amino acid to the tRNA which has the matching anticodon for that amino acid. When the amino acid binds to the tRNA molecule a high energy bond is created. The energy from this bond is used later on to bind the amino acids to the growing polypeptide chain during translation. |
| |
7.4.2 Outline the structure of ribosomes, including protein and RNA composition, large and small subunits, three tRNA binding sites and mRNA binding sites. |
|
Ribosomes have a particular structure. They are made up of proteins and ribosomal RNA. They have two subunits, one large the other small. On the surface of the ribosome there are three sites to which tRNA can bind to. However not more than two tRNA molecules can bind to the ribosome at one time. Also there is a site on the surface of the ribosome to which mRNA can bind to. |
| |
7.4.3 State that translation consists of initiation, elongation, translocation and termination. |
|
Translation consists of initiation, elongation, translocation and termination. |
| |
7.4.4 State that translation occurs in a 5'→3' direction. |
|
Translation occurs in a 5'→3' direction. |
| |
7.4.5 Draw and label a diagram showing the structure of a peptide bond between two amino acids. |
|

|
| |
7.4.6 Explain the process of translation, including ribosomes, polysomes, start codons and stop codons. |
|
Translation occurs in the cytoplasm. It starts off with the tRNA containing the matching anticodon for the start codon AUG binding to the small subunit of the ribosome. This tRNA carries the amino acid methionine and is always the first tRNA to bind to the P site. The small subunit of the ribosome then binds to the 5' end of the mRNA. This is because translation occurs in a 5'→3' direction. The small subunit will move along the mRNA until it reaches the start codon AUG. The large subunit of the ribosome can then binds to the small subunit. The next tRNA with the matching anticodon to the second codon on the mRNA binds to the A site of small subunit of the ribosome. The amino acids on the two tRNA molecules then form a peptide bond. Once this is done, the large subunit of the ribosome moves forward over the smaller one.The smaller subunit moves forward to join the larger subunit and as it does so the ribosome moves 3 nucleotides along the mRNA and the first tRNA is moved to the E site to be released. The second tRNA is now at the P site so that another tRNA with the matching anticodon can then bind to the A site. As this process continues the polypeptide is elongated. Once the ribosome reaches the stop codon on the mRNA translation will end as no tRNA will have a matching anticodon to the stop codon. The polypeptide is then released. Many ribosomes can translate the same mRNA at the same time. They will all move along the mRNA in a 5'→3' direction. These groups of ribosomes on a single mRNA are called polysomes. |
| |
7.4.7 State that free ribosomes synthesise proteins for use primarily within the cell, and that bound ribosomes synthesise proteins primarily for secretion or for lysosomes. |
|
Free ribosomes synthesise proteins for use primarily within the cell while bound ribosomes synthesise proteins primarily for secretion or for lysosomes. |
| |
7.5.1 Explain the four levels of protein structure, indicating the significance of each level. |
|
There are four levels of protein structure: Primary Structure: The primary structure of a protein is its amino acid sequence. This amino acid sequence is determined by the base sequence of the gene which codes for the protein. Secondary Structure: Secondary structures have α-helices and β-pleated sheets. These form as a result of hydrogen bonds between the peptide groups of the main chain. Therefore, proteins that contain secondary structures will have regions that are cylindrical (α-helices) and/or regions that are planar (β-pleated sheets). Tertiary Structure: The tertiary structure of a protein is its three-dimensional conformation which occurs as a result of the protein folding. This folding is stabilised by hydrogen bonds, hydrophobic interactions, ionic bonds and disulphide bridges. These intramolecular bonds form between the R groups of different amino acids. Quaternary Structure: A quaternary structure is formed when two or more polypeptide chains associate to form a single protein. An example is haemoglobin which consists of four polypeptide chains. In some cases, some proteins can have a non-polypeptide structure called a prosthetic group. These proteins are called conjugated proteins. The haem group in haemoglobin is a prosthetic group. |
| |
7.5.2 Outline the difference between fibrous and globular proteins, with reference to two examples of each protein type. |
|
Protein shape can be categorised as either fibrous or globular. Fibrous proteins tend to be elongated, physically tough and insoluble in water. Collagen found in the skin and keratin found in hair are examples of fibrous proteins. Globular proteins tend to be compact, rounded and water soluble. Haemoglobin and enzymes are examples of globular proteins. |
| |
7.5.3 Explain the significance of polar and non-polar amino acids. |
|
Amino acids have different R groups. Some of these R groups will be hydrophilic, making the amino acid polar, while others will be hydrophobic, making the amino acid non-polar. The distribution of the polar and non-polar amino acids in a protein influences the function and location of the protein within the body. Non-polar amino acids are found in the centre of water soluble proteins while the polar amino acids are found at the surface. Examples of how the distribution of non-polar and polar amino acids affect protein function and location: Controlling the position of proteins in membranes: The non-polar amino acids cause proteins to be embedded in membranes while polar amino acids cause portions of the proteins to protrude from the membrane. Creating hydrophilic channels through membranes: Polar amino acids are found inside membrane proteins and create a channel through which hydrophilic molecules can pass through. Specificity of active site in enzymes: If the amino acids in the active site of an enzyme are non-polar then it makes this active site specific to a non-polar substance. On the other hand, if the active site is made up of polar amino acids then the active site is specific to a polar substance. |
| |
7.5.4 State four functions of proteins, giving a named example of each. |
|
Function Example Structural Collagen strengthens bones, skin and tendons. Movement Myosin found in muscle fibers causes contraction of the muscle which results in movement. Transport Haemoglobin transports oxygen from the lungs to other tissues in the body. Defense Immunoglobulin acts as an antibody. |
| |
7.6.1 State that metabolic pathways consist of chains and cycles of enzyme-catalysed reactions. |
|
Metabolic pathways consist of chains and cycles of enzyme-catalysed reactions. |
| |
7.6.2 Describe the induced-fit model. |
|

Initially the substrate does not fit perfectly into the active site of the enzyme. When the substrate binds to the active site, this changes the shape of the active site and only then does it perfectly fit the substrate. As the substrate binds it changes the shape of the active site and this weakens the bonds in the substrate and therefore reduces the activation energy. This model is a more precise version of the lock and key one. The reason for this is that it explains why some enzymes can bind to many different substrates. If the shape of the active site changes when a substrate binds, this allows many different but similar substrates to bind to the one enzyme.
|
| |
7.6.3 Explain that enzymes lower the activation energy of the chemical reactions that they catalyse. |
|
Reactants of a chemical reaction need to gain energy before they can undergo the reaction. This required energy is called the activation energy of the reaction and it is needed to break bonds within the reactants. At a later stage in the reaction energy will be released as new bonds form. The majority of biological reactions are exothermic. In exothermic reactions the energy released by the new bonds formed is greater than the activation energy. In other words, the reaction releases energy. Enzymes make it easier for reactions to occur by decreasing the activation energy required in the reactions that they catalyse. |
| |
7.6.4 Explain the difference between competitive and non-competitive inhibition, with reference to one example each. |
|

Enzyme inhibitors are substances which inhibit enzyme activity. There are competitive enzyme inhibitors and non-competitive inhibitors. Competitive Inhibitors These are structurally similar to the substrate of the enzyme and bind to the active site. This means that when a competitive inhibitor binds to the active site of an enzyme, it prevents the substrate from binding to the active site. Only once the inhibitor has been released from the active site can the substrate bind. The inhibitor is called a competitive inhibitor as it competes with the substrate for the active site.

The effects of a competitive inhibitor can be reduced by increasing the substrate concentration. More substrate would successfully bind to the active site than inhibitor and therefore reducing the effect of the inhibition. The maximum rate of reaction or a level very close to the maximum rate of reaction can be reached.

An example of a competitive inhibitor is malonate. Malonate is structurally similar to the substrate succinate. Succinate is found in the Krebs cycle of aerobic respiration and binds to the active site of the dehydrogenase enzyme. Malonate can compete with succinate for the active site and in doing so it can prevent succinate from binding. Non-Competitive Inhibition These are not similar to the substrate and they do not bind to the active site of the enzyme. Instead they bind to a different site on the enzyme and change the conformation of the active site. The substrate may still be able to bind to the active site however the enzyme is not able to catalyse the reaction or can only do so at a slower rate.

Figure 7.6.5 - Non-competitive inhibition In the presence of a non-competitive inhibitor, increasing the substrate concentration cannot prevent the inhibitor from binding to the enzyme as the two bind to different sites. Therefore, no matter how high the concentration of substrate is, some of the enzymes will still be inhibited. The maximum rate of reaction will always be lower in the presence of a non-competitive inhibitor.

An example of a non-competitive inhibitor is ATP. When ATP accumulates it binds to a site other than the active site on the enzyme phosphofructokinase. In doing so it changes the enzyme conformation and lowers the rate of reaction so that less ATP is produced. |
| |
7.6.5 Explain the control of metabolic pathways by end-product inhibition, including the role of allosteric sites. |
|
Metabolic pathways are made up of many chemical reactions and these reactions are catalysed by enzymes. Often, the product of the last reaction in the pathway inhibits the enzyme that catalyses the first reaction of the pathway. This is called end-product inhibition and it involves non-competitive inhibitors. The product of the last reaction of the metabolic pathway will bind to a site other than the active site of the enzyme that catalyses the first reaction. This site is called the allosteric site. When it binds to the allosteric site it acts as non-competitive inhibitor and changes the conformation of the active site. Therefore, it makes the binding of the substrate to the enzyme unlikely. Once the inhibitor is released from the allosteric site, the active site returns to its original conformation and the substrate is able to bind again. There is a clear advantage in using end-product inhibition for controlling metabolic pathways. When there is an excess of end-product, the whole metabolic pathway is shut down as the end product inhibits the first enzyme of the pathway. Therefore less of the end product gets produced and by inhibiting the first enzyme it also prevents the formation of intermediates. When the levels of the end product decrease, the enzymes start to work again and the metabolic pathway is switched on. |
| |
8.1.1 State that oxidation involves the loss of electrons from an element, whereas reduction involves the gain of electrons and that oxidation frequently involves gaining oxygen or losing hydrogen, whereas reduction frequently involves losing oxygen or gaining hydrogen. |
|
Oxidation involves the loss of electrons from an element, whereas reduction involves the gain of electrons and that oxidation frequently involves gaining oxygen or losing hydrogen, whereas reduction frequently involves losing oxygen or gaining hydrogen. |
| |
8.1.2 Outline the process of glycolysis, including phosphorylation, lysis, oxidation and ATP formation. |
|

Step 1 - Glucose is phosphorylated. Two phosphate groups are added to glucose to form hexose biphosphate. These two phosphate groups are provided by two molecules of ATP. Step 2 - Lysis of hexose biphosphate. Hexose biphosphate splits into two molecules of triose phosphate. Step 3 - Each triose phosphate molecules is oxidised. Two atoms of hydrogen are removed from each molecule. The energy released by the oxidation is used to add another phosphate group to each molecule. This will result in two 3-carbon compounds, each carrying two phosphate groups. NAD+ is the hydrogen carrier that accepts the hydrogen atoms lost from each triose phosphate molecule. Step 4 - Two pyruvate molecules are formed by removing two phosphate groups from each molecule. These phosphate groups are given to ADP molecules and form ATP. Glycolysis occurs in the cytoplasm of cells. Two ATP molecules are used and 4 ATP molecules are produced. Therefore there is a net yield of two ATP molecules. Also, two NAD+ are converted into NADH + H+ during glycolysis.
|
| |
8.1.3 Draw and label a diagram showing the structure of a mitochondrion as seen in electron micrographs. |
|

|
| |
8.1.4 Explain aerobic respiration, including the link reaction, the Krebs cycle, the role of NADH + H+, the electron transport chain and the role of oxygen. |
|

Aerobic Respiration Glycolysis can take place without oxygen. This forms the anaerobic part of cell respiration and therefore is called anaerobic cell respiration. However, the pyruvate produced from glycolysis cannot be oxidised further without the presence of oxygen. The oxidisation of the pyruvate forms part of the aerobic respiration and therefore is called aerobic cell respiration. Aerobic respiration occurs in the mitochondria of cells. The first reaction to take place is the link reaction. The Link Reaction Mitochondria in cells take up the pyruvate which is formed from glycolysis in the cytoplasm. Once the pyruvate is in the mitochondrion, enzymes within the matrix of the mitochondrion remove hydrogen and carbon dioxide from the pyruvate. This is called oxidation (removal of hydrogen or addition of oxygen) and decarboxylation (removal of carbon dioxide). Therefore, the process is called oxidative decarboxylation. The hydrogen removed is accepted by NAD+. The link reaction results in the formation of an acetyl group. This acetyl group is then accepted by CoA and forms acetyl CoA.

Figure 8.1.3 - The link reaction The Krebs Cycle Step 1 - In the first stage of the Krebs cycle, the acetyl group from acetyl CoA is transferred to a four carbon compound. This forms a six carbon compound. Step 2 - This six carbon compound then undergoes decarboxylation (CO2 is removed) and oxidation (hydrogen is removed) to form a five carbon compound. The hydrogen is accepted by NAD+ and forms NADH + H+. Step 3 - The five carbon compound undergoes decarboxylation and oxidation (hydrogen is removed) again to form a four carbon compound. The hydrogen is accepted by NAD+ and forms NADH + H+. Step 4 - The four carbon compound then undergoes substrate-level phosphorylation and during this reaction it produces ATP. Oxidation also occurs twice (2 hydrogens are removed). The one hydrogen is accepted by NAD+ and forms NADH + H+. The other is accepted by FAD and forms FADH2. The four carbon compound is then ready to accept a new acetyl group and the cycle is repeated. The carbon dioxide that is removed in these reactions is a waste product and is excreted from the body. The oxidations release energy which is then stored by the carriers when they accept the hydrogen. This energy is then later on used by the electron transport chain to produce ATP.

The Electron Transport Chain Inside the inner membrane of the mitochondria there is a chain of electron carriers. This chain is called the electron transport chain. Electrons from the oxidative reactions in the earlier stages of cell respiration pass along the chain. NADH donates two electrons to the first carrier in the chain. These two electrons pass along the chain and release energy from one carrier to the next. At three locations along the chain, enough energy is released to produce ATP via ATP synthase. ATP synthase is an enzyme that is also found in the inner mitochondrial membrane. FADH2 also donates electrons but at a later stage than NADH. Also, enough energy is released at only two locations along the chain by electrons from FADH2. The ATP production relies on energy release by oxidation and it is therefore called oxidative phosphorylation.

Figure 8.1.5 - Electron transport chain The Role of Oxygen Oxygen is important for cell respiration as at the end of the electron transport chain, the electrons are donated to oxygen. This occurs in the matrix at the surface of the inner membrane. At the same time oxygen binds with hydrogen ions and forms water. If there is no oxygen then electrons can no longer pass through the electron transport chain and NADH + H+ can no longer be reconverted into NAD+. Eventually NAD+ in the mitochondrion runs out and therefore the link reaction and Krebs cycle no longer take place. |
| |
8.1.5 Explain oxidative phosphorylation in terms of chemiosmosis. |
|
 When electrons pass through the electron transport chain they release energy. This energy is then used to pump protons (H+) from the matrix across the inner mitochondrial membrane and into the space between the inner and outer mitochondrial membranes. The space between the inner and outer membranes has a small volume and therefore as the protons move across they create a concentration gradient very quickly. This process is called chemiosmosis. There is now a high concentration of protons in the space between the inner and outer membranes and a low concentration of protons in the matrix.

Figure 8.1.6 - Chemiosmosis Figure 8.1.6 shows the movement of protons from the matrix into the space between the inner and outer membranes. This creates a concentration gradient. The energy used to pump these protons across the inner membrane comes from the energy released by the electrons passing through the electron transport chain. The protons then move down the concentration gradient from the space between the inner and outer membranes back into the matrix. However, they can only move back across via an enzyme embedded in the inner membrane. This enzyme is called ATP synthase. The protons are transported back into the matrix through the channels of ATP synthase and as they do so they release energy. This energy is then used by ATP synthase to convert ADP into ATP. Since the electrons come from previous oxidation reactions of cell respiration and the ATP synthase catalyses the phosphorylation of ADP into ATP, this process is called oxidative phosphorylation. Chemiosmosis is necessary for oxidative phosphorylation to work.

Figure 8.1.7 shows the movement of protons down their concentration gradient. They can only travel through the inner membrane via ATP synthase and as they do so they release energy. This energy is used by ATP synthase to convert ADP into ATP. |
| |
8.1.6 Explain the relationship between the structure of the mitochondrion and its function. |
|
Matrix: Watery substance that contains ribosomes and many enzymes. These enzymes are vital for the link reaction and the Krebs cycle. Inner membrane: The electron transport chain and ATP synthase are found in this membrane. These are vital for oxidative phosphorylation. Space between inner and outer membranes: Small volume space into which protons are pumped into. Due to its small volume, a high concentration gradient can be reached very quickly. This is vital for chemiosmosis. Outer membrane: This membrane separates the contents of the mitochondrion from the rest of the cell. It creates a good environment for cell respiration. Cristae: These tubular projections of the inner membrane increase the surface area for oxidative phosphorylation. |
| |
8.2.1 Draw and label a diagram showing the structures of a chloroplast as seen in electron micrographs. |
|
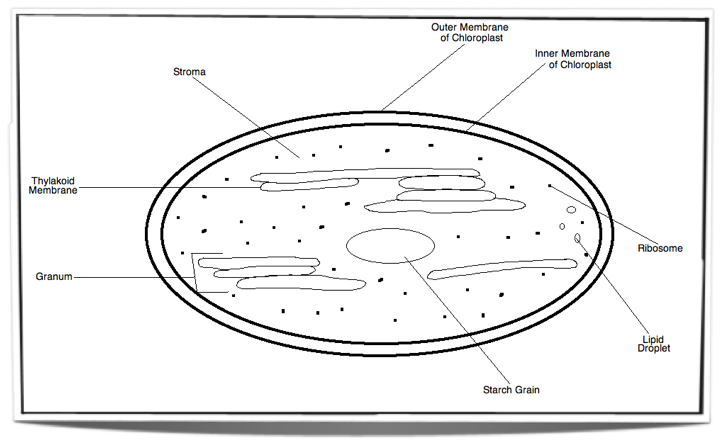
|
| |
8.2.2 State that photosynthesis consists of light-dependent and light-independent reactions. |
|
Photosynthesis consists of light-dependent and light-independent reactions. |
| |
8.2.3 Explain the light-dependent reactions. |
|
Photosynthesis occurs inside chloroplasts. Chloroplasts contain chlorophyll, a green pigment found inside the thylakoid membranes. These chlorophyll molecules are arranged in groups called photosystems. There are two types of photosystems, Photosystem II and Photosystem I. When a chlorophyll molecule absorbs light, the energy from this light raises an electron within the chlorophyll molecule to a higher energy state. The chlorophyll molecule is then said to be photoactivated. Excited electron anywhere within the photosystem are then passed on from one chlorophyll molecule to the next until they reach a special chlorophyll molecule at the reaction centre of the photosystem. This special chlorophyll molecule then passes on the excited electron to a chain of electron carriers. The light-dependent reactions starts within Photosystem II. When the excited electron reaches the special chlorophyll molecule at the reaction centre of Photosystem II it is passed on to the chain of electron carriers. This chain of electron carriers is found within the thylakoid membrane. As this excited electron passes from one carrier to the next it releases energy. This energy is used to pump protons (hydrogen ions) across the thylakoid membrane and into the space within the thylakoids. This forms a proton gradient. The protons can travel back across the membrane, down the concentration gradient, however to do so they must pass through ATP synthase. ATP synthase is located in the thylakoid membrane and it uses the energy released from the movement of protons down their concentration gradient to synthesise ATP from ADP and inorganic phosphate. The synthesis of ATP in this manner is called non-cyclic photophosphorylation (uses the energy of excited electrons from photosystem II) . The electrons from the chain of electron carriers are then accepted by Photosystem I. These electrons replace electrons previously lost from Photosystem I. Photosystem I then absorbs light and becomes photoactivated. The electrons become excited again as they are raised to a higher energy state. These excited electrons then pass along a short chain of electron carriers and are eventually used to reduce NADP+ in the stroma. NADP+ accepts two excited electrons from the chain of carriers and one H+ ion from the stroma to form NADPH. If the light intensity is not a limiting factor, there will usually be a shortage of NADP+ as NADPH accumulates within the stroma (see light independent reaction). NADP+ is needed for the normal flow of electrons in the thylakoid membranes as it is the final electron acceptor. If NADP+ is not available then the normal flow of electrons is inhibited. However, there is an alternative pathway for ATP production in this case and it is called cyclic photophosphorylation. It begins with Photosystem I absorbing light and becoming photoactivated. The excited electrons from Photosystem I are then passed on to a chain of electron carriers between Photosystem I and II. These electrons travel along the chain of carriers back to Photosystem I and as they do so they cause the pumping of protons across the thylakoid membrane and therefore create a proton gradient. As explained previously, the protons move back across the thylakoid membrane through ATP synthase and as they do so, ATP is produced. Therefore, ATP can be produced even when there is a shortage of NADP+. In addition to producing NADPH, the light dependent reactions also produce oxygen as a waste product. When the special chlorophyll molecule at the reaction centre passes on the electrons to the chain of electron carriers, it becomes positively charged. With the aid of an enzyme at the reaction centre, water molecules within the thylakoid space are split. Oxygen and H+ ions are formed as a result and the electrons from the splitting of these water molecules are given to chlorophyll. The oxygen is then excreted as a waste product. This splitting of water molecules is called photolysis as it only occurs in the presence of light. |
| |
8.2.4 Explain photophosphorylation in terms of chemiosmosis. |
|
Photophosphorylation is the production of ATP using the energy of sunlight. Photophosphorylation is made possible as a result of chemiosmosis. Chemiosmosis is the movement of ions across a selectively permeable membrane, down their concentration gradient. During photosynthesis, light is absorbed by chlorophyll molecules. Electrons within these molecules are then raised to a higher energy state. These electrons then travel through Photosystem II, a chain of electron carriers and Photosystem I. As the electrons travel through the chain of electron carriers, they release energy. This energy is used to pump hydrogen ions across the thylakoid membrane and into the space within the thylakoid. A concentration gradient of hydrogen ions forms within this space. These then move back across the thylakoid membrane, down their concentration gradient through ATP synthase. ATP synthase uses the energy released from the movement of hydrogen ions down their concentration gradient to synthesise ATP from ADP and inorganic phosphate. |
| |
8.2.5 Explain the light-independant reactions. |
|
The light-independant reactions of photosynthesis occur in the stroma of the chloroplast and involve the conversion of carbon dioxide and other compounds into glucose. The light-independent reactions can be split into three stages, these are carbon fixation, the reduction reactions and finally the regeneration of ribulose bisphosphate. Collectively these stages are known as the Calvin Cycle. During carbon fixation, carbon dioxide in the stroma (which enters the chloroplast by diffusion) reacts with a five-carbon sugar called ribulose bisphosphate (RuBP) to form a six-carbon compound. This reaction is catalysed by an enzyme called ribulose bisphosphate carboxylase (large amounts present within the stroma), otherwise known as rubisco. As soon as the six-carbon compound is formed, it splits to form two molecules of glycerate 3-phosphate. Glycerate 3-phosphate is then used in the reduction reactions. Glycerate 3-phosphate is reduced during the reduction reactions to a three-carbon sugar called triose phosphate. Energy and hydrogen is needed for the reduction and these are supplied by ATP and NADPH + H+ (both produced during light-dependent reactions) respectively. Two triose phosphate molecules can then react together to form glucose phosphate. The condensation of many molecules of glucose phosphate forms starch which is the form of carbohydrate stored in plants. However, out of six triose phosphates produced during the reduction reactions, only one will be used to synthesise glucose phosphate. The five remaining triose phosphates will be used to regenerate RuBP. The regeneration of RuBP is essential for carbon fixation to continue. Five triose phosphate molecules will undergo a series of reactions requiring energy from ATP, to form three molecules of RuBP. RuBP is therefore consumed and produced during the light-independent reactions and therefore these reactions form a cycle which is named the Calvin cycle. |
| |
8.2.6 Explain the relationship between the structure of the chloroplast and its function. |
|
The stroma - Contains many enzymes, including rubisco, which are important for the reactions of the Calvin cycle. The thylakoids - Have a large surface area for light absorption and the space within them allows rapid accumulation of protons. |
| |
8.2.7 Explain the relationship between the action spectrum and the absorption spectrum of photosynthetic pigments in green plants. |
|
The action spectrum of photosynthesis is a graph showing the rate of photosynthesis for each wavelength of light. The rate of photosynthesis will not be the same for every wavelength of light. The rate of photosynthesis is the least with green-yellow light (525 nm-625 nm). Red-orange light (625nm-700nm) shows a good rate of photosynthesis however the best rate of photosynthesis is seen with violet-blue light (400nm-525nm). An absorption spectrum is a graph showing the percentage of light absorbed by pigments within the chloroplast, for each wavelength of light. An example is the absorption spectrum of chlorophyll a and b. The best absorption is seen with violet-blue light. There is also good absorption with red-orange light. However most of the green-yellow light is reflected and therefore not absorbed. This wavelength of light shows the least absorption. As we can see, there is a close relationship between the action spectrum and absorption spectrum of photosynthesis. There are many different types of photosynthetic pigments which will absorb light best at different wavelengths. However the most abundant photosynthetic pigment in plants is chlorophyll and therefore the rate of photosynthesis will be the greatest at wavelengths of light best absorbed by chlorophyll (400nm-525nm corresponding to violet-blue light). Very little light is absorbed by chlorophyll at wavelengths of light between 525nm and 625 (green-yellow light) so the rate of photosynthesis will be the least within this range. However, there are other pigments that are able to absorb green-yellow light such as carotene. Even though these are present in small amounts they allow a low rate of photosynthesis to occur at wavelengths of light that chlorophyll cannot absorb. |
| |
8.2.8 Explain the concept of limiting factors in photosynthesis, with reference to light intensity, temperature and concentration of carbon dioxide. |
|
A limiting factor is a factor that controls a process. Light intensity, temperature and carbon dioxide concentration are all factors which can control the rate of photosynthesis. Usually, only one of these factors will be the limiting factor in a plant at a certain time. This is the factor which is the furthest from its optimum level at a particular point in time. If we change the limiting factor the rate of photosynthesis will change but changes to the other factors will have no effect on the rate. If the levels of the limiting factor increase so that this factor is no longer the furthest from its optimum level, the limiting factor will change to the factor which is at that point in time, the furthest from its optimum level. For example, at night the limiting factor is likely to be the light intensity as this will be the furthest from its optimum level. During the day, the limiting factor is likely to switch to the temperature or the carbon dioxide concentration as the light intensity increases. So how can these factors have an effect on the rate of photosynthesis? Lets start off with the light intensity. When the light intensity is poor, there is a shortage of ATP and NADPH, as these are products from the light dependent reactions. Without these products the light independent reactions can't occur as glycerate 3-phosphate cannot be reduced. Therefore a shortage of these products will limit the rate of photosynthesis. When the carbon dioxide concentration is low, the amount of glycerate 3-phosphate produced is limited as carbon dioxide is needed for its production and therefore the rate of photosynthesis is affected. Finally, many enzymes are involved during the process of photosynthesis. At low temperatures these enzymes work slower. At high temperatures the enzymes no longer work effectively. This affects the rate of the reactions in the Calvin cycle and therefore the rate of photosynthesis will be affected. |
| |
9.1.1 Draw and label plan diagrams to show the distribution of tissues in the stem and leaf of a dicotyledonous plant. |
|

|
| |
9.1.2 Outline three differences between the structures of dicotyledonous and monocotyledonous plants. |
|
Monocotyledons Dicotyledons One cotyledon Two cotyledons Vascular bundles are randomly spread through out the stem Vascular bundles are arranged in a ring Parallel venation in leaves Net-like venation in leaves Floral organs are in multiples of 3 Floral organs are in multiples of 4 or 5 New roots form from the stem New roots branch from pre-existing roots |
| |
9.1.3 Explain the relationship between the distribution of tissues in the leaf and the functions of these tissues. |
|
Upper epidermis - Consists of a single layer of cells found on the upper surface of the leaf. It is covered by a thick waxy cuticle. The main function of the upper epidermis is water conservation. It prevents the loss of water from the upper surface where the light intensity and heat are the greatest. Palisade mesophyll - Consists of tightly packed cylindrical cells. This tissue contains many chloroplasts as it is the main photosynthetic tissue. It is found on the upper half of the leaf (upper surface) where the light intensity is the greatest. Spongy mesophyll - Made up of loosely packed cells. This tissue is found in the lower half of the leaf (lower surface) and has few chloroplasts. It provides gas exchange (CO2 uptake and O2release) and therefore needs to be close to the stomata found in the lower epidermis. Vascular tissue - Consists of xylem and phloem which are found in the veins of the leaf. The veins in the leaf are positioned in the middle so that all the cells are in close contact with the vascular tissue. The xylem consists of xylem vessels (dead structure) which are long and tubular and transports water into the leaf to replace the water that has been lost through transpiration. The phloem is made up of living cells with pores in between them. It transports the products of photosynthesis out of the leaf. |
| |
9.1.4 Identify modifications of roots, stems and leaves for different functions: bulbs, stem tubers, storage roots and tendrils. |
|

Bulbs: These are modified leaf bases which serve as food storage and thereby enable the plant to survive adverse conditions.These leaf bases may look like scales or they may extend over and encircle the centre of the bulb (onion). At the base of the bulb, a modified stem can be seen. Roots grow from the underside of the base while the new stems and leaves arise from the upper side of the base. An example of a bulb is an onion bulb.

Stem Tubers: These are modified stems which serve as food storage. The stem extends into the ground and forms enlarged, swollen structures which we call stem tubers. Stem tubers are used to store nutrients and therefore allow the plant to survive winter as well as other adverse conditions. They also serve as a mean of asexual reproduction as new plants develop from these stem tubers. An example of a stem tuber is a potato.

Storage Roots: These are modified roots which serve as food storage. They also allow the plant to survive adverse conditions. An example of a storage root is a carrot.

Tendrils: These are modified leaves. They are slim and provide attachment as well as support. In doing so they allow plants to climb upwards. They will rotate in the air until they reach a solid structure to which they can attach to. An example of plants with tendrils are grape vines.
|
| |
9.1.5 State that dicotyledonous plants have apical and lateral meristems. |
|
Dicotyledonous plants have apical and lateral meristems. |
| |
9.1.6 Compare growth due to apical and lateral meristems in dicotyledonous plants. |
|
The plant meristem is a type of tissue found at several locations on plants. This tissue is composed of cells which are totipotent. This means that these cells are able to divide and make all the types of cells of that particular plant at any given time. Meristem tissue allows continuous growth and the formation of new organs. Apical meristems are found at the tips of roots and shoots. The apical meristem is responsible for the elongation of roots and stems. It allows the stem to grow taller and the roots to increase in length. Also, the shoot apical meristem allows the formation of new leaves and flowers. The growth in height of the stem is important for photosynthesis while the lengthening of the roots is important for the plant to anchor deep into the soil and it is also vital for the uptake of water and nutrients found in deeper soil layers. The growth taking place at apical meristems is called primary growth. In addition, plants also grow by increasing the diameter of their stems and roots. This is called secondary growth and is a result of cell devision in the lateral meristems. It allows extra xylem and phloem tissue production and it also provides stability for the plant to grow taller. |
| |
9.1.7 Explain the role of auxin in phototropism as an example of the control of plant growth. |
|
Tropisms are directional movement responses which occur due to external environmental stimuli. The direction of the stimulus affects the direction of movement. Tropisms can either be negative or positive. Positive tropisms are the directional movement towards the stimulus while negative tropisms are the directional movement away from the stimulus. Examples of stimuli causing tropisms in plants are gravity and light. Roots will grow towards gravity while the plant shoot will grow upwards in the opposite direction. The directional movement of plants in response to light is called phototropism. As seen with gravity, the plant's roots will grow away from the light, into the soil (negative phototropism) while the plant shoot will grow towards the light (positive phototropism). Positive phototropism seen at the tips of plant shoots is made possible due to plant hormones called auxins. Auxins are produced at the tips of plant shoots and then translocate to the darker side of the shoot tip and stem which is receiving less light. This translocation is made possible via auxin efflux carriers which are unevenly distributed in the plant tissue. Once auxins reach the shaded side of the plant, they cause the elongation of cells so that the shaded side grows faster than the brighter side, thereby promoting the bending of the plant shoot towards the light. Auxins do so by binding to auxin receptors on cells. The binding of auxin causes the transcription of certain genes within those cells and therefore the production of specific proteins which affect growth. Auxins allow the expelling of protons (hydrogen ions) into the cell walls of the cells on the shaded side, decreasing the pH inside the cells and in doing so activate specific enzymes which break down cellulose microfibrils within the cell wall. This loosens the cell wall and allows cell elongation. So to conclude, auxins are very important in the control of plant growth towards the light and thereby allow the plant to increase its rate of photosynthesis. |
| |
9.2.1 Outline how the root system provides a large surface area for mineral ion and water uptake by means of branching and root hairs. |
|
Plant roots are very important for water and mineral ion absorption as well as the anchoring of the plant into the ground. Germination causes the embryonic root to break through the seed coat and start growing down into the soil. A whole root system then develops by the branching of this embryonic root into new roots, increasing the surface area for absorption. The surface area is further increased by the branching of root hairs from these roots. |
| |
9.2.2 List ways in which mineral ions in the soil move to the root. |
|
Mineral ions in the soil move to roots via fungal hyphae (mutualism), mass flow of water in the soil carrying ions and the diffusion of mineral ions. |
| |
9.2.3 Explain the process of mineral ion absorption from the soil into roots by active transport. |
|
The concentration of mineral ions inside the plant's roots is a lot higher than that found in the soil. Therefore, mineral ions have to be transported into the roots via active transport. Protein pumps exist in the plasma membranes of root cells. There are many types of these protein pumps for the absorption of many different mineral ions. Active transport requires ATP production by mitochondria (aerobic cell respiration, oxygen is needed) and therefore the root cells also contain many mitochondria. The branching of roots and the formation of root hairs increases the surface area for the absorption of mineral ions by active transport. |
| |
9.2.4 State that terrestrial plants support themselves by means of thickened cellulose, cell turgor and lignified xylem. |
|
Terrestrial plants support themselves by means of thickened cellulose, cell turgor and lignified xylem. |
| |
9.2.5 Define transpiration. |
|
Transpiration is the loss of water vapour from the leaves and stems of plants. |
| |
9.2.6 Explain how water is carried by the transpiration stream, including the structure of xylem vessels, transpiration pull, cohesion, adhesion and evaporation. |
|
Once water has been taken up by the roots it is pulled upwards into the leaves where it then evaporates. This flow of water from the roots to the leaves is called the transpiration stream. This transpiration stream occurs in xylem vessels and the movement of water is passive. Mature xylem vessels are long dead structures made up of cells arranged from end to end. The cell walls between the adjacent xylem cells are broken down and the cytoplasmic content dies to form a continuous tube. The cells also lack a plasma membrane which allows water to enter the vessels freely. In addition, they also contain pores in the outer cell walls which allows the movement of water out of the vessels and into the surrounding cells of leaves. The outer cell walls contain thickenings which resemble spirals or rings impregnated with lignin which makes the vessels strong and able to withstand low pressures. Low pressure (suction) is created in the xylem vessels when water is pulled out of the transpiration stream via evaporation of water vapour from the spongy mesophyll cell walls in the leaves. Heat from the environment is necessary as it provides the energy required for the evaporation of water. The low pressure causes more water from the roots to be pulled upwards through the xylem tubes, this is called transpiration pull. Transpiration pull works due to the cohesion of water molecules. Hydrogen bonds form between the water molecules allowing the formation of columns of water which are not easily broken by the low pressure. In addition, adhesion also plays a role in maintaining transpiration pull. The water molecules adhere to the walls of the xylem vessels preventing the columns of water from breaking. So to conclude, the structure of xylem vessels, transpiration pull, cohesion, adhesion and evaporation are all important in the carrying of water by the transpiration stream. |
| |
9.2.7 State that guard cells can regulate transpiration by opening and closing stomata. |
|
Guard cells can regulate transpiration by opening and closing stomata. |
| |
9.2.8 State that the plant hormone abscisic acid causes the closing of stomata. |
|
The plant hormone abscisic acid causes the closing of stomata. |
| |
9.2.9 Explain how the abiotic factors light, temperature, wind and humidity, affect the rate of transpiration in a typical terrestrial plant. |
|
Four abiotic factors affect the rate of transpiration in a typical terrestrial plant: Light - The rate of transpiration is much greater when light is available as the stomata close in the dark. Humidity - Water diffuses out of the leaf, down its concentration gradient, from a high concentration gradient inside the leaf to a lower concentration gradient in the air. The lower concentration gradient in the air is vital for transpiration. Humidity is the water vapour in the air, therefore a rise in humidity means a larger concentration of water vapour in the air and results in a decrease in transpiration rate. Temperature - As temperature rises, so does the rate of transpiration. This is because heat is vital for the evaporation of water vapour from the cell walls of spongy mesophyll cells. A rise in temperature leads to an increase in the evaporation rate thereby increasing transpiration rate. Higher temperatures also increase the rate of diffusion between air spaces inside the leaf and the air outside. Finally, an increase in temperature causes a reduction in humidity in the air outside the leaf which causes an increase in concentration gradient and therefore an increase in transpiration rate. Wind - Wind increases the transpiration rate by removing the humidity around the leaf produced by transpiration. |
| |
9.2.10 Outline four adaptations of xerophytes that help to reduce transpiration. |
|
1) Reduced surface area of the plant - reduced leaves such as spines in cacti (modified leaves) 2) Thick waxy cuticle covering the epidermis 3) Reduced numbers of stomata 4) Water storage tissues in roots, leaves and stems 5) CAM physiology - Stomata open during the evening/night instead of during the day (when the temperature is at its highest) as the transpiration rate will be lower during cooler hours. |
| |
9.2.11 Outline the role of phloem in active translocation of sugars (sucrose) and amino acids from source (photosynthetic tissue and storage organs) to sink (fruits, seeds, roots). |
|
Phloem tissue transports sugars and amino acids from sources which include photosynthetic tissue (leaves and stems) and storage organs, to sinks which include the fruits, seeds and roots of the plant. This transport is known as active translocation and requires energy. |
| |
9.3.1 Draw and label a diagram showing the structure of a dicotyledonous animal-pollinated flower. |
|

|
| |
9.3.2 Distinguish between pollination, fertilization and seed dispersal. |
|
Pollination - The process of pollen transfer from an anther to a stigma. Fertilization - The fusion of a male gamete with a female gamete inside the ovule. This forms a zygote. Seed dispersal - The movement/transport of seeds away from the plant. Fruits which develop from fertilised ovules, function as a mean of seed dispersal. |
| |
9.3.3 Draw and label a diagram showing the external and internal structure of a named dicotyledonous seed. |
|

|
| |
9.3.4 Explain the conditions needed for the germination of a typical seed. |
|
Germination is the emerging and growth of an embryonic plant from a seed. It requires certain conditions, such as water, heat and oxygen. If conditions are not favourable then the seed may remain dormant. This way the seed can survive adverse conditions and only start to germinate when conditions become more favourable. Water is needed to rehydrate the cells of the seed. This is vital for the activation of certain enzymes which start the metabolism of the seed. Without water the embryo root and shoot are not able to grow. Also water causes the seed to swell and this leads to the bursting of the seed coat which enables the plant to emerge from the seed. In addition, heat is needed for germination as the enzyme activity inside the seed depends on it. However, appropriate temperatures are needed. If it is too hot or too cold the enzyme activity will be too low for germination. Therefore, seeds usually remain dormant if heat conditions are not favourable. Finally, oxygen is needed for metabolism. It is used in aerobic cell respiration to provide the energy for the growth of the plant until the first leaves emerge. Once the leaves emerge, photosynthesis can then provide the energy needed for growth.
|
| |
9.3.5 Outline the metabolic processes during germination of a starchy seed. |
|
The absorption of water is followed by the formation of gibberellin in the embryo's cotyledon. This stimulates the production of amylase which catalyses the breakdown of starch into maltose. This maltose then diffuses to the embryo for energy release and growth. |
| |
9.3.6 Explain how flowering is controlled in long-day and short-day plants, including the role of phytochrome. |
|
Flowering in long-day and short-day plants is controlled by a pigment called phytochrome. This pigment exists in two forms, Pr and Pfr, which can be converted into each other. The Pr form is the inacctive form and absorbs red light with a wavelength of 660 nm. When Pr absorbs red light it is rappidly converted to the active Pfr form. The Pfr form absorbs far red light with a wavelength of 730 nm and then rapidly converts to the Pr form again in day light. However, normal day light contains more light of wavelength of 660 nm rather than 730 nm. This means that in normal day light, there is more Pfr than there is Pr. However the Pr form is the more stable of the two and therefore during the dark hours Pfr is slowly converted back into Pr. The amount of Pfr remaining after the dark nights is most likely the way plants can time the legth of dark periods. If the dark period is short, this means there will be a lot of Pfr as not much of it will have converted back into Pr. If the dark period is long, less Pfr will be available as most of it will have been converted back into Pr. Furthermore, Pfr promotes flowering in long day plants and inhibits flowering in short-day plants. Therfore, during long dark periods (autumn-winter) short day plants will flower as most of the Pfr has been converted back into Pr in the morning. On the other hand, long-day plants flower in the shorter dark periods (spring-summer) as enough Pfr remains in the morning to promote flowering. |
| |
10.1.1 Describe the behaviour of the chromosomes in the phases of meiosis. |
|

Two divisions occure during meiosis, these are termed meiosis I and meiosis II. Each division involves the four stages of prophase, metaphase, anaphase and telophase. Meiosis I Prophase I - Chromosomes coil up tightly and become visible under a light microscope
- Homologous chromosomes pair up and crossing over occures (the point of cross over is known as the chiasma)
- Nuclear membrane disintgrates and the centrioles travel to the poles of the cell
Metaphase I - Microtubules form a spindle and the spindle fibers attach to the centromeres of the chromosomes
- Pairs of homologous chromosomes align along the equator
Anaphase I - Spindle fibers shorten pulling paired homologous chromosomes in opposite directions
- Paired homologous chromosomes are seperated and pulled to opposite poles so that each pole contains one chromosome of each pair.
Telophase I - A nuclear membrane forms around the chromosomes at each pole and chromosomes uncoil
- The cell undergoes cytokinesis to form two daughter cells
- Forms two haploid cells
- At the end of telophase I the cells may enter a short interphase period or proceed directly to meiosis II
- DNA is not replicated
Meiosis II
Prophase II - Chromosomes coil up again
- Centrioles move to the cell poles
- Nuclear membrane disintergrates
Metaphase II - Spindle fibers attach to the the centromeres
- Chromosomes align along the equator
Anaphase II - Spindle fibers shorten
- Centromeres split
- Chromatids of each chromosome travel to opposite poles
Telophase II - Nuclear membrane forms around the chromatids at each pole, once the membrane is formed, each chromatid is then called a chromosome.
- Both cells undergo cytokinesis to form four cells
- Chromosomes uncoil
- Nucleoli form
|
| |
10.1.2 Outline the formation of chiasmata in the process of crossing over |
|
In prophase I the four sister chromatids of a pair of homologous chromosomes become tightly linked in a process called synapsis. A cut is made in the DNA molecule of one of the chromatids. Following this another cut is made at the same point in the DNA molecule of a non-sister chromatid. The DNA of the one chromatid binds to the DNA of the non-sister chromatid. Paternal and maternal chromosomes can then exchange genetic material. This is called crossing over. Once crossing over is finnished the homologous chromosomes are no longer tightly linked however the connection between the non-sister chromatids remains, forming an X - shaped structure called a chiasma. The chiasma links homologous chromosome pairs together and remains until late metaphase I. |
| |
10.1.3 Explain how meiosis results in an effectively infinite genetic variety in gametes through crossing over in prophase I and random orientation in metaphase I. |
|
Two processes result in the infinite genetic variety in gametes. These are crossing over in prophase I and the random orientation of chromosomes in metaphase I. Crossing over is important for genetic variety as it allows the exchange of genetic material between the maternal and paternal chromosomes. This forms chromatids with new combinations of alleles (recombination of linked genes). The chromatids which have a combination of allele different to that of either parent are called recombinants. It is also important to note that crossing over occures at a random point and more than one chiasma can form per homologous pair. This means that meiosis can result in almost an infinite amount of genetic variety. The random orientation of homologous chromosomes at the equator in metaphase I also plays a vital role in genetic variety. Since the homologous pairs of chromosomes are orientated randomly at the equator, either maternal or paternal homolgue can orient towards either pole. The number of possible orientations is equal to 2 raised to the power of the number of chromosome pairs. For example, for a haploid number of n, 2n is the number of possible outcomes. Humans have a haploid number of 23. 223 gives a value of over 8 million. This means that there are over 8 million possible combinations just through the radom orientation of the homologous chromosmes. If we add the effects of crossing over, the number of combinations increases even further. Therfore, these two processes allow infinit genetic variety in gametes. |
| |
10.1.4 State Mendel's law of independent assortment. |
|
Allele pairs seperate independently during gamete formation which means that the transmission of traits to offspring are independent to one another. |
| |
10.1.5 Explain the relationship between Mendel's law of independent assortment and meiosis. |
|
During metaphase I of meiosis the homologous pairs of chromosomes align along the equator. The orientation of the chromosomes is random. This means that when the pairs of homologous chromosomes move to opposite poles during anaphase I, either chromosome can end up at either pole. This depends on which way the pair is facing (occurs randomly). Also, which ever way the pair is facing does not affect which way the other homologous chromosome pairs are facing. This is known as idenpendent orientation and forms the basis of Mendel's law of independent assortment. Unlinked genes are found on different chromosomes so when the homologous chromosome pairs seperate it allows the formation of daughter cells with random assortemnets of chromosomes and alleles. |
| |
10.2.1 Calculate and predict the genotypic and phenotypic ratio of offspring of dihybrid crosses involving unlinked autosomal genes. |
|

A dihybrid cross is a cross between first generation offspring of two individuals which have two different characteristics. These two characteristics are controled by two genes. We can use dihybrid crosses to calculate and predict the genotypic and phenotypic ratio of offspring involving unlinked autosomal genes. For example: Let's say we cross two cats with two different characteristics such as fur colour and fur length. For this example we will be using the alleles as follows: - S = allele for long fur
- s = allele for short fur
- B = allele for black fur
- b = allele for brown fur
|
| |
10.2.2 Distinguish between autosomes and sex chromosomes. |
|
Sex chromosomes are the ones that determine your gender. These are X and Y (XX in females, XY in males). Autosomal chromosomes are the remaining chromosomes which are not sex chromosomes. There are 22 pairs of these in humans. This means that there is a total of 23 pairs of chromosomes in humans (22 autosomal pairs + 1 sex chromosome pair = 23 pairs of chromosomes). |
| |
10.2.3 Explain how crossing over between non-sister chromatids of a homologous pair in prophase I can result in an exchange of alleles. |
|

|
| |
10.2.3 Define linkage group. |
|
Linkage group: A pair or set of genes on a chromosome which tend to be inherited together. |
| |
10.3.1 Define polygenic inheritance |
|
the inheritance of a characteristic which is controlled by more than one gene |
| |
10.3.2 explain that polygenic inheritance can contribute to continuous variation using 2 examples, one of which must be human skin color. |
|
if a true breeding pea-combed fowl is crossed with a true breeding rose-combed fowl the F1 all display a walnut comb. if 2 of these are bred, the f2 shows all of these combs along with a single comb |
| |
11.1.1 Describe the process of blood clotting. |
|
as |
| |
11.1.2 Outline the principle of challenge and response, clonal selection and memory cells as the basis of immunity. |
|
asd |
| |
11.1.3 Define active and passive immunity. |
|
asd |
| |
11.1.4 Explain anitbody production. |
|
asd |
| |
11.1.5 Describe the production of monoclonal antibodies and their use in the diagnosis and in treatment. |
|
sd |
| |
11.1.6 Explain the principle of vaccination |
|
asd |
| |
11.1.7 Discuss the benefits and dangers of vaccination |
|
asd |
| |
11.2.1 State the roles of bones, ligaments, muscles, tendons and nerves in human movement. |
|
asd |
| |
11.2.2 Label a diagram of the human elbow joint, including cartilage, synovial fluid, joint capsule, named bones and antagonistic muscles |
|
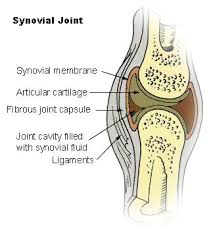
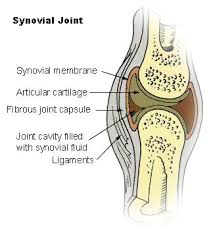
|
| |